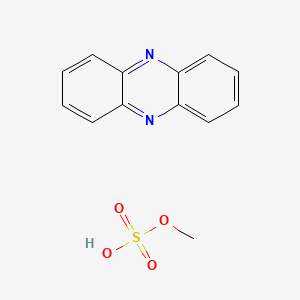
Methyl hydrogen sulfate;phenazine
- Click on QUICK INQUIRY to receive a quote from our team of experts.
- With the quality product at a COMPETITIVE price, you can focus more on your research.
Overview
Description
Methyl hydrogen sulfate: and phenazine are two distinct chemical compounds with unique properties and applications. Methyl hydrogen sulfate is an organosulfur compound with the formula CH₃HSO₄. It is a derivative of sulfuric acid and is used in various chemical processes. Phenazine, on the other hand, is a nitrogen-containing heterocyclic compound with the formula C₁₂H₈N₂. It is known for its vibrant pigmentation and diverse biological activities, including antimicrobial and antitumor properties .
Preparation Methods
Methyl Hydrogen Sulfate
Methyl hydrogen sulfate can be synthesized through the esterification of methanol with sulfuric acid. The reaction is typically carried out under controlled conditions to ensure the formation of the desired product:
CH3OH+H2SO4→CH3HSO4+H2O
Phenazine
Phenazine can be synthesized through various methods, including the Wohl–Aue method, Beirut method, and condensation of 1,2-diaminobenzenes with 2-carbon units. Industrial production often involves the oxidative cyclization of 1,2-diaminobenzene or diphenylamines under specific conditions .
Chemical Reactions Analysis
Methyl Hydrogen Sulfate
Methyl hydrogen sulfate undergoes hydrolysis to produce methanol and sulfuric acid. It can also participate in esterification reactions to form various organic sulfates.
Phenazine
Phenazine undergoes a variety of chemical reactions, including:
Oxidation: Phenazine can be oxidized to form phenazine N-oxide.
Reduction: Reduction of phenazine can yield dihydrophenazine derivatives.
Substitution: Phenazine can undergo electrophilic substitution reactions, such as nitration and halogenation
Scientific Research Applications
Methyl Hydrogen Sulfate
Methyl hydrogen sulfate is used in organic synthesis as a methylating agent. It is also employed in the production of detergents and surfactants.
Phenazine
Phenazine and its derivatives have a wide range of applications in scientific research:
Chemistry: Used as intermediates in the synthesis of dyes and pigments.
Biology: Studied for their role in bacterial metabolism and biofilm formation.
Medicine: Investigated for their antimicrobial, antitumor, and antioxidant properties.
Industry: Utilized in the development of electronic materials and sensors
Mechanism of Action
Methyl Hydrogen Sulfate
Methyl hydrogen sulfate acts as a methylating agent, transferring a methyl group to various substrates. This reaction is facilitated by the presence of sulfuric acid, which acts as a catalyst.
Phenazine
Phenazine exerts its effects through multiple mechanisms:
Electron Shuttling: Phenazine serves as an electron shuttle, facilitating redox reactions in bacterial cells.
Gene Regulation: Phenazine can modulate gene expression, influencing bacterial behavior and biofilm formation.
Reactive Oxygen Species: Phenazine generates reactive oxygen species, contributing to its antimicrobial and antitumor activities
Comparison with Similar Compounds
Methyl Hydrogen Sulfate
Similar compounds include ethyl hydrogen sulfate and propyl hydrogen sulfate, which differ in the alkyl group attached to the sulfate moiety.
Phenazine
Phenazine is structurally related to other nitrogen-containing heterocycles such as pyrazine and quinoxaline. These compounds share similar chemical reactivity but differ in their biological activities and applications .
Conclusion
Methyl hydrogen sulfate and phenazine are versatile compounds with significant roles in various fields of science and industry. Their unique properties and diverse applications make them valuable tools for researchers and industrial chemists alike.
Properties
IUPAC Name |
methyl hydrogen sulfate;phenazine |
Source
|
---|---|---|
Details | Computed by Lexichem TK 2.7.0 (PubChem release 2021.05.07) | |
Source | PubChem | |
URL | https://pubchem.ncbi.nlm.nih.gov | |
Description | Data deposited in or computed by PubChem | |
InChI |
InChI=1S/C12H8N2.CH4O4S/c1-2-6-10-9(5-1)13-11-7-3-4-8-12(11)14-10;1-5-6(2,3)4/h1-8H;1H3,(H,2,3,4) |
Source
|
Details | Computed by InChI 1.0.6 (PubChem release 2021.05.07) | |
Source | PubChem | |
URL | https://pubchem.ncbi.nlm.nih.gov | |
Description | Data deposited in or computed by PubChem | |
InChI Key |
HCAONSFIGUBAPW-UHFFFAOYSA-N |
Source
|
Details | Computed by InChI 1.0.6 (PubChem release 2021.05.07) | |
Source | PubChem | |
URL | https://pubchem.ncbi.nlm.nih.gov | |
Description | Data deposited in or computed by PubChem | |
Canonical SMILES |
COS(=O)(=O)O.C1=CC=C2C(=C1)N=C3C=CC=CC3=N2 |
Source
|
Details | Computed by OEChem 2.3.0 (PubChem release 2021.05.07) | |
Source | PubChem | |
URL | https://pubchem.ncbi.nlm.nih.gov | |
Description | Data deposited in or computed by PubChem | |
Molecular Formula |
C13H12N2O4S |
Source
|
Details | Computed by PubChem 2.1 (PubChem release 2021.05.07) | |
Source | PubChem | |
URL | https://pubchem.ncbi.nlm.nih.gov | |
Description | Data deposited in or computed by PubChem | |
Molecular Weight |
292.31 g/mol |
Source
|
Details | Computed by PubChem 2.1 (PubChem release 2021.05.07) | |
Source | PubChem | |
URL | https://pubchem.ncbi.nlm.nih.gov | |
Description | Data deposited in or computed by PubChem | |
CAS No. |
2360-48-7 |
Source
|
Record name | Sulfuric acid, monomethyl ester, compd. with phenazine | |
Source | CAS Common Chemistry | |
URL | https://commonchemistry.cas.org/detail?cas_rn=2360-48-7 | |
Description | CAS Common Chemistry is an open community resource for accessing chemical information. Nearly 500,000 chemical substances from CAS REGISTRY cover areas of community interest, including common and frequently regulated chemicals, and those relevant to high school and undergraduate chemistry classes. This chemical information, curated by our expert scientists, is provided in alignment with our mission as a division of the American Chemical Society. | |
Explanation | The data from CAS Common Chemistry is provided under a CC-BY-NC 4.0 license, unless otherwise stated. | |
Disclaimer and Information on In-Vitro Research Products
Please be aware that all articles and product information presented on BenchChem are intended solely for informational purposes. The products available for purchase on BenchChem are specifically designed for in-vitro studies, which are conducted outside of living organisms. In-vitro studies, derived from the Latin term "in glass," involve experiments performed in controlled laboratory settings using cells or tissues. It is important to note that these products are not categorized as medicines or drugs, and they have not received approval from the FDA for the prevention, treatment, or cure of any medical condition, ailment, or disease. We must emphasize that any form of bodily introduction of these products into humans or animals is strictly prohibited by law. It is essential to adhere to these guidelines to ensure compliance with legal and ethical standards in research and experimentation.