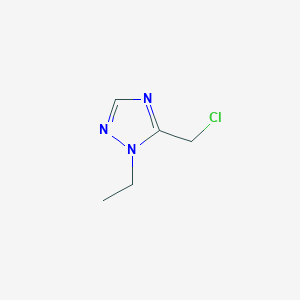
5-(chloromethyl)-1-ethyl-1H-1,2,4-triazole
- Click on QUICK INQUIRY to receive a quote from our team of experts.
- With the quality product at a COMPETITIVE price, you can focus more on your research.
Overview
Description
5-(Chloromethyl)-1-ethyl-1H-1,2,4-triazole: is a heterocyclic organic compound that features a triazole ring substituted with a chloromethyl group at the 5-position and an ethyl group at the 1-position
Preparation Methods
Synthetic Routes and Reaction Conditions: The synthesis of 5-(chloromethyl)-1-ethyl-1H-1,2,4-triazole typically involves the following steps:
Formation of the Triazole Ring: The triazole ring can be synthesized through a cyclization reaction involving hydrazine and an appropriate nitrile or amidine precursor.
Chloromethylation: The chloromethyl group can be introduced via a chloromethylation reaction, often using formaldehyde and hydrochloric acid in the presence of a catalyst.
Industrial Production Methods: Industrial production of this compound may involve continuous flow processes to ensure high yield and purity. The use of automated reactors and precise control of reaction conditions can optimize the production process.
Chemical Reactions Analysis
Types of Reactions:
Substitution Reactions: The chloromethyl group can undergo nucleophilic substitution reactions, where the chlorine atom is replaced by various nucleophiles.
Oxidation and Reduction: The triazole ring can participate in oxidation and reduction reactions, altering the electronic properties of the compound.
Addition Reactions: The compound can undergo addition reactions with electrophiles or nucleophiles, depending on the reaction conditions.
Common Reagents and Conditions:
Nucleophilic Substitution: Common reagents include sodium azide, potassium cyanide, and various amines. Reactions are typically carried out in polar solvents such as dimethyl sulfoxide (DMSO) or acetonitrile.
Oxidation: Oxidizing agents such as hydrogen peroxide or potassium permanganate can be used.
Reduction: Reducing agents like sodium borohydride or lithium aluminum hydride are commonly employed.
Major Products Formed:
Substitution Products: Depending on the nucleophile used, products can include azides, nitriles, or amines.
Oxidation Products: Oxidation can lead to the formation of triazole N-oxides.
Reduction Products: Reduction can yield various reduced triazole derivatives.
Scientific Research Applications
Chemistry:
Catalysis: 5-(Chloromethyl)-1-ethyl-1H-1,2,4-triazole can be used as a ligand in coordination chemistry, facilitating various catalytic processes.
Synthesis of Complex Molecules: It serves as a building block for the synthesis of more complex heterocyclic compounds.
Biology and Medicine:
Antimicrobial Agents: The compound has potential as an antimicrobial agent due to its ability to disrupt microbial cell processes.
Pharmaceuticals: It can be used in the development of drugs targeting specific enzymes or receptors.
Industry:
Materials Science: The compound can be incorporated into polymers to enhance their properties, such as thermal stability and resistance to degradation.
Agriculture: It may be used in the synthesis of agrochemicals, including pesticides and herbicides.
Mechanism of Action
Molecular Targets and Pathways: The mechanism of action of 5-(chloromethyl)-1-ethyl-1H-1,2,4-triazole involves its interaction with specific molecular targets, such as enzymes or receptors. The chloromethyl group can form covalent bonds with nucleophilic sites on proteins, leading to inhibition or modification of their activity. The triazole ring can also participate in hydrogen bonding and π-π interactions, further influencing the compound’s biological activity.
Comparison with Similar Compounds
5-(Chloromethyl)-1-methyl-1H-1,2,4-triazole: Similar structure but with a methyl group instead of an ethyl group.
5-(Bromomethyl)-1-ethyl-1H-1,2,4-triazole: Similar structure but with a bromomethyl group instead of a chloromethyl group.
1-Ethyl-1H-1,2,4-triazole: Lacks the chloromethyl group, providing a basis for comparison of reactivity and properties.
Uniqueness: 5-(Chloromethyl)-1-ethyl-1H-1,2,4-triazole is unique due to the presence of both the chloromethyl and ethyl groups, which confer specific reactivity and properties. The chloromethyl group enhances its potential for nucleophilic substitution reactions, while the ethyl group influences its solubility and steric properties.
Properties
IUPAC Name |
5-(chloromethyl)-1-ethyl-1,2,4-triazole |
Source
|
---|---|---|
Details | Computed by LexiChem 2.6.6 (PubChem release 2019.06.18) | |
Source | PubChem | |
URL | https://pubchem.ncbi.nlm.nih.gov | |
Description | Data deposited in or computed by PubChem | |
InChI |
InChI=1S/C5H8ClN3/c1-2-9-5(3-6)7-4-8-9/h4H,2-3H2,1H3 |
Source
|
Details | Computed by InChI 1.0.5 (PubChem release 2019.06.18) | |
Source | PubChem | |
URL | https://pubchem.ncbi.nlm.nih.gov | |
Description | Data deposited in or computed by PubChem | |
InChI Key |
KLWMDJXWPBWTLD-UHFFFAOYSA-N |
Source
|
Details | Computed by InChI 1.0.5 (PubChem release 2019.06.18) | |
Source | PubChem | |
URL | https://pubchem.ncbi.nlm.nih.gov | |
Description | Data deposited in or computed by PubChem | |
Canonical SMILES |
CCN1C(=NC=N1)CCl |
Source
|
Details | Computed by OEChem 2.1.5 (PubChem release 2019.06.18) | |
Source | PubChem | |
URL | https://pubchem.ncbi.nlm.nih.gov | |
Description | Data deposited in or computed by PubChem | |
Molecular Formula |
C5H8ClN3 |
Source
|
Details | Computed by PubChem 2.1 (PubChem release 2019.06.18) | |
Source | PubChem | |
URL | https://pubchem.ncbi.nlm.nih.gov | |
Description | Data deposited in or computed by PubChem | |
Molecular Weight |
145.59 g/mol |
Source
|
Details | Computed by PubChem 2.1 (PubChem release 2021.05.07) | |
Source | PubChem | |
URL | https://pubchem.ncbi.nlm.nih.gov | |
Description | Data deposited in or computed by PubChem | |
Disclaimer and Information on In-Vitro Research Products
Please be aware that all articles and product information presented on BenchChem are intended solely for informational purposes. The products available for purchase on BenchChem are specifically designed for in-vitro studies, which are conducted outside of living organisms. In-vitro studies, derived from the Latin term "in glass," involve experiments performed in controlled laboratory settings using cells or tissues. It is important to note that these products are not categorized as medicines or drugs, and they have not received approval from the FDA for the prevention, treatment, or cure of any medical condition, ailment, or disease. We must emphasize that any form of bodily introduction of these products into humans or animals is strictly prohibited by law. It is essential to adhere to these guidelines to ensure compliance with legal and ethical standards in research and experimentation.