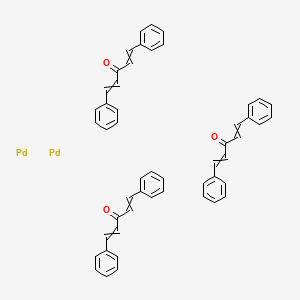
Tris(dibenzylideneacetone)dipalladium(0)
Overview
Description
Tris(dibenzylideneacetone)dipalladium(0) is an organopalladium compound, often referred to by its chemical formula, C51H42O3Pd2. This compound is a complex of palladium(0) with dibenzylideneacetone ligands. It appears as a dark-purple or brown solid and is modestly soluble in organic solvents . Tris(dibenzylideneacetone)dipalladium(0) is widely used as a homogeneous catalyst in organic synthesis due to the ease with which its dibenzylideneacetone ligands can be displaced .
Mechanism of Action
Target of Action
Tris(dibenzylideneacetone) dipalladium(0), also known as Pd2(dba)3, is an organopalladium compound . The primary targets of this compound are various biochemical reactions where it acts as a catalyst . It is known to facilitate oxidative addition reactions . In the context of cancer treatment, Tris(dibenzylideneacetone) dipalladium(0) has been found to target the STAT3 signaling pathway .
Mode of Action
The compound is a complex of palladium(0) with dibenzylideneacetone (dba). The Pd(0) centers are bound to the alkene parts of the dba ligands . It is known for its high reactivity and ability to facilitate oxidative addition reactions . In cancer treatment, it abrogates the STAT3 signaling pathway by elevating the expression of SHP2 .
Biochemical Pathways
Pd2(dba)3 is used as a catalyst for several reactions including Suzuki cross-coupling, Heck coupling, arylation, Buchwald-Hartwig amination, and fluorination . These reactions are crucial in various biochemical pathways. In the context of cancer treatment, it affects the STAT3 signaling pathway .
Pharmacokinetics
It is known that the compound is a dark-purple/brown solid, which is modestly soluble in organic solvents . This solubility could potentially affect its absorption, distribution, metabolism, and excretion (ADME) properties, and thus its bioavailability.
Result of Action
As a catalyst, Pd2(dba)3 facilitates various biochemical reactions, leading to the production of desired compounds . In cancer treatment, Tris(dibenzylideneacetone) dipalladium(0) has been found to inhibit cell proliferation, migration, invasion, and regress tumor metastasis .
Action Environment
The action of Tris(dibenzylideneacetone) dipalladium(0) can be influenced by various environmental factors. For instance, its solubility in organic solvents suggests that the presence and type of solvent can affect its action, efficacy, and stability.
Biochemical Analysis
Biochemical Properties
Tris(dibenzylideneacetone) dipalladium(0) has been found to interact with several biomolecules, including enzymes and proteins. For instance, it has been shown to inhibit the STAT3 signaling pathway in hepatocellular carcinoma and multiple myeloma cancer cells . This inhibition is achieved by elevating the expression of SHP2 .
Cellular Effects
Tris(dibenzylideneacetone) dipalladium(0) has significant effects on various types of cells and cellular processes. It has been shown to abrogate tumor progression in hepatocellular carcinoma and multiple myeloma preclinical models by regulating the STAT3 signaling pathway .
Molecular Mechanism
At the molecular level, Tris(dibenzylideneacetone) dipalladium(0) exerts its effects through binding interactions with biomolecules and changes in gene expression. It inhibits the STAT3 signaling pathway, which is a major oncogenic transcription factor that is constitutively activated in many types of human cancers .
Temporal Effects in Laboratory Settings
It is known that it has significant antiproliferative activity against melanoma cells .
Dosage Effects in Animal Models
The effects of Tris(dibenzylideneacetone) dipalladium(0) vary with different dosages in animal models. It has been found to have activity against B16 murine and A375 human melanoma in vivo .
Metabolic Pathways
It is known to inhibit several signaling pathways including activation of mitogen-activated protein kinase, Akt, Stat-3, and S6 kinase activation .
Preparation Methods
Tris(dibenzylideneacetone)dipalladium(0) was first reported in 1970. It is synthesized by reacting dibenzylideneacetone with sodium tetrachloropalladate . The reaction typically involves the following steps:
- Dissolve dibenzylideneacetone in an organic solvent such as ethanol.
- Add sodium tetrachloropalladate to the solution.
- Stir the mixture under an inert atmosphere (e.g., nitrogen) at elevated temperatures.
- Recrystallize the product from chloroform to obtain the pure compound .
Chemical Reactions Analysis
Tris(dibenzylideneacetone)dipalladium(0) is known for its high reactivity and ability to facilitate various palladium-catalyzed reactions. Some of the key reactions it undergoes include:
Oxidative Addition: This reaction involves the addition of a substrate to the palladium center, forming a new palladium-substrate bond.
Reductive Elimination: This reaction involves the elimination of a product from the palladium center, regenerating the palladium(0) species.
Substitution: The dibenzylideneacetone ligands can be easily displaced by other ligands, making it a versatile catalyst
Common reagents and conditions used in these reactions include:
Suzuki Coupling: Uses aryl halides and boronic acids in the presence of a base.
Heck Coupling: Involves aryl halides and alkenes under basic conditions.
Buchwald-Hartwig Amination: Uses aryl halides and amines with a base
Scientific Research Applications
Tris(dibenzylideneacetone)dipalladium(0) has a wide range of applications in scientific research, including:
Chemistry: It is used as a catalyst in various coupling reactions, such as Suzuki, Heck, and Buchwald-Hartwig amination
Biology: It has been studied for its potential use in the synthesis of biologically active compounds.
Medicine: Research has explored its use in the development of new pharmaceuticals and therapeutic agents.
Industry: It is used in the production of fine chemicals and materials, including polymers and nanomaterials.
Comparison with Similar Compounds
Tris(dibenzylideneacetone)dipalladium(0) is unique due to its high reactivity and versatility as a catalyst. Similar compounds include:
Bis(dibenzylideneacetone)palladium(0): Another palladium(0) complex with similar catalytic properties.
Tetrakis(triphenylphosphine)palladium(0): A palladium(0) complex with triphenylphosphine ligands, used in similar catalytic applications.
Palladium(II) acetate: A palladium(II) compound used as a precursor in various palladium-catalyzed reactions.
Tris(dibenzylideneacetone)dipalladium(0) stands out due to its ease of ligand displacement and high catalytic efficiency in a wide range of reactions .
Properties
IUPAC Name |
1,5-diphenylpenta-1,4-dien-3-one;palladium | |
---|---|---|
Details | Computed by Lexichem TK 2.7.0 (PubChem release 2021.05.07) | |
Source | PubChem | |
URL | https://pubchem.ncbi.nlm.nih.gov | |
Description | Data deposited in or computed by PubChem | |
InChI |
InChI=1S/3C17H14O.2Pd/c3*18-17(13-11-15-7-3-1-4-8-15)14-12-16-9-5-2-6-10-16;;/h3*1-14H;; | |
Details | Computed by InChI 1.0.6 (PubChem release 2021.05.07) | |
Source | PubChem | |
URL | https://pubchem.ncbi.nlm.nih.gov | |
Description | Data deposited in or computed by PubChem | |
InChI Key |
CYPYTURSJDMMMP-UHFFFAOYSA-N | |
Details | Computed by InChI 1.0.6 (PubChem release 2021.05.07) | |
Source | PubChem | |
URL | https://pubchem.ncbi.nlm.nih.gov | |
Description | Data deposited in or computed by PubChem | |
Canonical SMILES |
C1=CC=C(C=C1)C=CC(=O)C=CC2=CC=CC=C2.C1=CC=C(C=C1)C=CC(=O)C=CC2=CC=CC=C2.C1=CC=C(C=C1)C=CC(=O)C=CC2=CC=CC=C2.[Pd].[Pd] | |
Details | Computed by OEChem 2.3.0 (PubChem release 2021.05.07) | |
Source | PubChem | |
URL | https://pubchem.ncbi.nlm.nih.gov | |
Description | Data deposited in or computed by PubChem | |
Molecular Formula |
C51H42O3Pd2 | |
Details | Computed by PubChem 2.1 (PubChem release 2021.05.07) | |
Source | PubChem | |
URL | https://pubchem.ncbi.nlm.nih.gov | |
Description | Data deposited in or computed by PubChem | |
Molecular Weight |
915.7 g/mol | |
Details | Computed by PubChem 2.1 (PubChem release 2021.05.07) | |
Source | PubChem | |
URL | https://pubchem.ncbi.nlm.nih.gov | |
Description | Data deposited in or computed by PubChem | |
Retrosynthesis Analysis
AI-Powered Synthesis Planning: Our tool employs the Template_relevance Pistachio, Template_relevance Bkms_metabolic, Template_relevance Pistachio_ringbreaker, Template_relevance Reaxys, Template_relevance Reaxys_biocatalysis model, leveraging a vast database of chemical reactions to predict feasible synthetic routes.
One-Step Synthesis Focus: Specifically designed for one-step synthesis, it provides concise and direct routes for your target compounds, streamlining the synthesis process.
Accurate Predictions: Utilizing the extensive PISTACHIO, BKMS_METABOLIC, PISTACHIO_RINGBREAKER, REAXYS, REAXYS_BIOCATALYSIS database, our tool offers high-accuracy predictions, reflecting the latest in chemical research and data.
Strategy Settings
Precursor scoring | Relevance Heuristic |
---|---|
Min. plausibility | 0.01 |
Model | Template_relevance |
Template Set | Pistachio/Bkms_metabolic/Pistachio_ringbreaker/Reaxys/Reaxys_biocatalysis |
Top-N result to add to graph | 6 |
Feasible Synthetic Routes
Q1: What is the molecular formula and weight of tris(dibenzylideneacetone)dipalladium(0)?
A1: The molecular formula of tris(dibenzylideneacetone)dipalladium(0) is C51H42O3Pd2, and its molecular weight is 915.72 g/mol. []
Q2: What is the structure of tris(dibenzylideneacetone)dipalladium(0)?
A2: Tris(dibenzylideneacetone)dipalladium(0) features a "paddlewheel" structure with two palladium atoms bridged by three dibenzylideneacetone (dba) ligands. Each palladium atom coordinates to two double bonds of dba ligands, exhibiting a distorted square planar geometry. []
Q3: What are the typical spectroscopic characteristics of tris(dibenzylideneacetone)dipalladium(0)?
A3: Tris(dibenzylideneacetone)dipalladium(0) exhibits a characteristic deep purple to black color. It shows distinct signals in NMR (1H and 13C) spectroscopy corresponding to the dba ligands. Additionally, it displays characteristic absorption bands in UV-Vis spectroscopy, notably a strong absorption in the visible range (λmax = 528 nm). [, ]
Q4: How stable is tris(dibenzylideneacetone)dipalladium(0) in air and solution?
A4: Tris(dibenzylideneacetone)dipalladium(0) is moderately air stable in the solid state but slowly decomposes in solution to metallic palladium and dibenzylideneacetone. [] This decomposition is particularly noticeable in the presence of coordinating solvents or upon exposure to light and heat.
Q5: Why is tris(dibenzylideneacetone)dipalladium(0) widely used in organic synthesis?
A5: Tris(dibenzylideneacetone)dipalladium(0) is a valuable palladium(0) source that readily undergoes oxidative addition, enabling its use as a catalyst or precatalyst in various palladium-catalyzed cross-coupling reactions. [] These reactions, including Suzuki, Stille, Heck, and Sonogashira couplings, are essential for forming carbon-carbon bonds and have widespread applications in organic synthesis, materials science, and medicinal chemistry.
Q6: Can you provide some examples of reactions where tris(dibenzylideneacetone)dipalladium(0) is used as a catalyst?
A6: Tris(dibenzylideneacetone)dipalladium(0) catalyzes a wide range of reactions, including:
- Suzuki-Miyaura coupling: Coupling of aryl or vinyl halides with organoboron compounds to form biaryl or alkene products. [, , ]
- Stille coupling: Coupling of aryl or vinyl halides with organotin compounds to form biaryl or alkene products. [, , ]
- Heck reaction: Coupling of aryl or vinyl halides with alkenes to form substituted alkenes. []
- Sonogashira coupling: Coupling of aryl or vinyl halides with terminal alkynes to form arylalkynes or enynes. []
- Buchwald-Hartwig amination: Coupling of aryl halides with amines to form arylamines. [, ]
Q7: What are the advantages of using tris(dibenzylideneacetone)dipalladium(0) as a catalyst?
A7: Tris(dibenzylideneacetone)dipalladium(0) offers several advantages as a catalyst:
Q8: How does tris(dibenzylideneacetone)dipalladium(0) activate in catalytic reactions?
A8: Tris(dibenzylideneacetone)dipalladium(0) typically requires activation in situ by dissociating one or more dba ligands to generate coordinatively unsaturated palladium(0) species. These species can then undergo oxidative addition with the substrate, initiating the catalytic cycle. [] The activation can be facilitated by external ligands like phosphines, amines, or carbenes, which stabilize the active palladium species and enhance catalytic activity and selectivity.
Q9: How does the choice of solvent affect reactions catalyzed by tris(dibenzylideneacetone)dipalladium(0)?
A9: The solvent choice can significantly impact the reactivity and selectivity of tris(dibenzylideneacetone)dipalladium(0) catalyzed reactions. Polar aprotic solvents, such as tetrahydrofuran, toluene, dioxane, and dimethylformamide, are commonly used. The solvent can influence the solubility of reactants and catalysts, the rate of ligand dissociation, and the stability of reaction intermediates, ultimately affecting the reaction outcome. [, , ]
Q10: What are some challenges associated with using tris(dibenzylideneacetone)dipalladium(0) as a catalyst?
A10: Despite its numerous advantages, some challenges exist when using tris(dibenzylideneacetone)dipalladium(0):
Q11: Does tris(dibenzylideneacetone)dipalladium(0) have applications beyond catalysis?
A11: Yes, besides its extensive use in catalytic reactions, tris(dibenzylideneacetone)dipalladium(0) has also been explored in other areas:
- Nanoparticle synthesis: Acts as a precursor for preparing palladium nanoparticles with controlled size and morphology. [, , ]
Q12: What are the safety concerns associated with handling tris(dibenzylideneacetone)dipalladium(0)?
A12: Tris(dibenzylideneacetone)dipalladium(0) is harmful and requires careful handling.
Q13: How should tris(dibenzylideneacetone)dipalladium(0) be disposed of properly?
A13: Tris(dibenzylideneacetone)dipalladium(0) should be disposed of following local regulations and guidelines for hazardous waste. Do not release into the environment.
Q14: What are some ongoing research areas related to tris(dibenzylideneacetone)dipalladium(0)?
A14: Research on tris(dibenzylideneacetone)dipalladium(0) continues to expand its applications:
- Developing more efficient and selective catalytic systems: Exploring new ligands and reaction conditions to improve catalytic performance. []
- Understanding the mechanism of action: Investigating the reaction pathways and intermediates involved in tris(dibenzylideneacetone)dipalladium(0) catalyzed reactions. []
Disclaimer and Information on In-Vitro Research Products
Please be aware that all articles and product information presented on BenchChem are intended solely for informational purposes. The products available for purchase on BenchChem are specifically designed for in-vitro studies, which are conducted outside of living organisms. In-vitro studies, derived from the Latin term "in glass," involve experiments performed in controlled laboratory settings using cells or tissues. It is important to note that these products are not categorized as medicines or drugs, and they have not received approval from the FDA for the prevention, treatment, or cure of any medical condition, ailment, or disease. We must emphasize that any form of bodily introduction of these products into humans or animals is strictly prohibited by law. It is essential to adhere to these guidelines to ensure compliance with legal and ethical standards in research and experimentation.