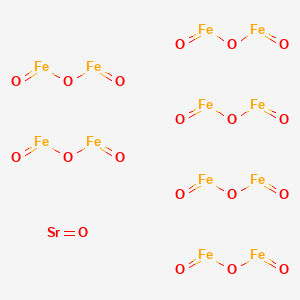
Strontium ferrite
- Click on QUICK INQUIRY to receive a quote from our team of experts.
- With the quality product at a COMPETITIVE price, you can focus more on your research.
Overview
Description
Strontium ferrite, chemically known as strontium hexaferrite, is a permanent magnetic material with a hexagonal crystal structure. Its chemical formula is SrFe({12})O({19}). This compound is widely recognized for its cost-effectiveness, excellent magnetic properties, and outstanding corrosion resistance. This compound is extensively used in various industrial applications, including permanent magnets, electronic devices, and magnetic recording media .
Mechanism of Action
Target of Action
Oxo(oxoferriooxy)iron;oxostrontium, also known as Strontium ferrite, is a complex compound that primarily targets the iron and strontium ions in various biochemical processes . The role of these ions is crucial in a multitude of cellular functions, including vital processes such as mitochondrial respiration, gene regulation, and DNA synthesis or repair .
Mode of Action
The interaction of Oxo(oxoferriooxy)iron;oxostrontium with its targets involves a series of redox reactions. The compound’s mode of action is characterized by the transition between the Fe(II) and Fe(III) valence states, forming a complex network of biochemical interactions . This includes a tight interplay of biotic and abiotic reactions, which play a fundamental role in environmental biogeochemistry .
Biochemical Pathways
The biochemical pathways affected by Oxo(oxoferriooxy)iron;oxostrontium involve the metabolism and biogeochemical cycling of iron . The compound influences the oxidation and reduction processes and the biochemical mechanisms associated with electron transport . The downstream effects of these pathways include the generation of ATP, DNA synthesis and repair, and oxygen transport .
Pharmacokinetics
The ADME (Absorption, Distribution, Metabolism, and Excretion) properties of Oxo(oxoferriooxy)iron;oxostrontium are yet to be fully understood. It is known that the compound’s bioavailability is influenced by its interaction with iron and strontium ions, and its role in various biochemical processes .
Result of Action
The molecular and cellular effects of Oxo(oxoferriooxy)iron;oxostrontium’s action are primarily seen in its influence on iron metabolism and biogeochemical cycling. The compound’s interaction with iron and strontium ions affects a range of cellular functions, including ATP generation, DNA synthesis and repair, and oxygen transport .
Action Environment
The action, efficacy, and stability of Oxo(oxoferriooxy)iron;oxostrontium are influenced by various environmental factors. These include the presence of other ions, the pH of the environment, and the presence of other compounds. For instance, the equatorial ligand effect has been shown to have a significant impact on the oxidative properties of an iron(V)-oxo species, affecting orbital interactions and enhancing their reactivities with substrates .
Preparation Methods
Synthetic Routes and Reaction Conditions: Strontium ferrite can be synthesized through several methods, including the sol-gel method, solid-state reaction, and mechanochemical process. The sol-gel method involves the preparation of a gel from a solution containing strontium and iron precursors, followed by calcination at high temperatures to form the ferrite structure . The solid-state reaction method involves mixing strontium carbonate (SrCO({3})) and iron oxide (Fe({2})O(_{3})) in a specific molar ratio, followed by heating at high temperatures to induce the formation of this compound . The mechanochemical process involves the use of mechanical energy to induce chemical reactions between strontium and iron precursors .
Industrial Production Methods: In industrial settings, this compound is typically produced using the solid-state reaction method due to its simplicity and cost-effectiveness. The process involves mixing strontium carbonate and iron oxide in a ball mill to achieve a homogeneous mixture. The mixture is then calcined at temperatures ranging from 1000°C to 1200°C to form this compound .
Chemical Reactions Analysis
Types of Reactions: Strontium ferrite undergoes various chemical reactions, including oxidation, reduction, and substitution. These reactions can alter its magnetic and structural properties.
Common Reagents and Conditions:
Oxidation: this compound can be oxidized in the presence of oxygen at high temperatures, leading to changes in its magnetic properties.
Reduction: Reduction reactions can be carried out using hydrogen gas or other reducing agents to modify the oxidation state of iron within the ferrite structure.
Major Products Formed: The major products formed from these reactions include modified ferrite compounds with altered magnetic and structural properties. For example, copper-doped this compound exhibits enhanced microwave absorption properties .
Scientific Research Applications
Strontium ferrite has a wide range of scientific research applications across various fields:
Chemistry:
- Used as a catalyst in various chemical reactions due to its magnetic properties and stability.
Biology:
- Employed in magnetic separation techniques for isolating biological molecules and cells.
Medicine:
- Investigated for its potential use in targeted drug delivery systems and magnetic hyperthermia for cancer treatment .
Industry:
Comparison with Similar Compounds
- Barium ferrite (BaFe({12})O({19}))
- Calcium ferrite (CaFe({12})O({19}))
- Manganese ferrite (MnFe({2})O({4}))
- Nickel ferrite (NiFe({2})O({4}))
Strontium ferrite stands out among these compounds due to its balance of cost, magnetic properties, and corrosion resistance, making it a preferred choice for various industrial applications.
Biological Activity
Strontium ferrite (SrFe12O19) is a magnetic ceramic material known for its applications in various fields, including electronics, catalysis, and biomedical engineering. Recent research has highlighted its biological activity, particularly in antibacterial and antifungal applications, as well as its potential use in environmental remediation. This article reviews the biological activity of this compound, focusing on its mechanisms of action, applications, and relevant case studies.
1. Overview of this compound
This compound is a hard magnetic material that exhibits excellent stability and resistance to corrosion. Its unique properties make it suitable for various applications, including:
- Electromagnetic devices : Used in permanent magnets.
- Catalysis : Acts as a catalyst in chemical reactions.
- Biomedical applications : Explored for drug delivery and antimicrobial agents.
2. Mechanisms of Biological Activity
This compound exhibits significant biological activity through several mechanisms:
- Antibacterial Properties : Studies have shown that this compound can inhibit the growth of various bacterial strains. The antibacterial mechanism is primarily attributed to the release of metal ions, which disrupt bacterial cell membranes and metabolic processes.
- Antifungal Activity : Similar to its antibacterial effects, this compound has demonstrated antifungal properties against different fungal species. The efficacy varies depending on the strain and the concentration used.
3.1 this compound Graphene Composite (SF@GOC)
A recent study investigated the biological activity of a novel composite made from this compound and graphene oxide (SF@GOC). The findings are summarized in Table 1.
Property | Value/Observation |
---|---|
Antibacterial Activity (E. coli) | 96.2% reduction |
Antifungal Activity (Candida spp.) | Varies by strain |
Degradation of Pollutants (Eosin-Y) | 96.2% degradation |
Degradation of Pollutants (Orange II) | 98.7% degradation |
The study revealed that SF@GOC exhibited enhanced antibacterial and antifungal activities compared to graphene oxide alone. The bactericidal mechanism was found to be effective against both gram-negative bacteria, indicating its potential for environmental remediation and biomedical applications .
3.2 Rubber Composites with this compound
Another study focused on this compound's incorporation into natural rubber composites. The research highlighted:
- Increased Mechanical Strength : The addition of this compound improved the tensile strength of rubber composites.
- Magnetic Properties : Enhanced remanent magnetic induction was observed with increased this compound content.
This study suggests that this compound can enhance not only mechanical properties but also provide antimicrobial benefits in composite materials used in medical applications .
4. Applications in Environmental Remediation
This compound's ability to degrade hazardous pollutants has been explored extensively. The SF@GOC composite has been particularly effective in breaking down dyes such as Eosin-Y and Orange II under mild reaction conditions, showcasing its potential as a sustainable catalyst for environmental cleanup .
5. Conclusion
This compound demonstrates significant biological activity with promising applications in various fields, including antibacterial and antifungal treatments, environmental remediation, and material science. Ongoing research continues to unveil its potential benefits and mechanisms of action, paving the way for innovative uses in healthcare and environmental technologies.
Properties
IUPAC Name |
oxo(oxoferriooxy)iron;oxostrontium |
Source
|
---|---|---|
Source | PubChem | |
URL | https://pubchem.ncbi.nlm.nih.gov | |
Description | Data deposited in or computed by PubChem | |
InChI |
InChI=1S/12Fe.19O.Sr |
Source
|
Source | PubChem | |
URL | https://pubchem.ncbi.nlm.nih.gov | |
Description | Data deposited in or computed by PubChem | |
InChI Key |
MUDURESJCZWWBG-UHFFFAOYSA-N |
Source
|
Source | PubChem | |
URL | https://pubchem.ncbi.nlm.nih.gov | |
Description | Data deposited in or computed by PubChem | |
Canonical SMILES |
O=[Fe]O[Fe]=O.O=[Fe]O[Fe]=O.O=[Fe]O[Fe]=O.O=[Fe]O[Fe]=O.O=[Fe]O[Fe]=O.O=[Fe]O[Fe]=O.O=[Sr] |
Source
|
Source | PubChem | |
URL | https://pubchem.ncbi.nlm.nih.gov | |
Description | Data deposited in or computed by PubChem | |
Molecular Formula |
Fe12O19Sr |
Source
|
Source | PubChem | |
URL | https://pubchem.ncbi.nlm.nih.gov | |
Description | Data deposited in or computed by PubChem | |
Molecular Weight |
1061.7 g/mol |
Source
|
Source | PubChem | |
URL | https://pubchem.ncbi.nlm.nih.gov | |
Description | Data deposited in or computed by PubChem | |
Q1: What is the chemical formula and crystal structure of strontium ferrite?
A1: this compound has the chemical formula SrFe12O19. It crystallizes in a magnetoplumbite hexagonal structure. []
Q2: What are the typical magnetic properties of this compound?
A2: this compound exhibits hard magnetic properties, characterized by high coercivity and remanence. The specific saturation magnetization can reach up to 71 emu/g, and coercivity values up to 6000 Oe have been reported. [, ]
Q3: What synthesis methods are commonly employed for this compound?
A4: Common synthesis routes include the conventional ceramic method, sol-gel techniques, co-precipitation, and ultrasonic spray pyrolysis. [, , , , ]
Q4: Can this compound be synthesized from industrial by-products?
A5: Yes, researchers have successfully synthesized this compound using magnetic iron species extracted from electrocoagulation by-products. []
Q5: What is the role of calcination temperature in this compound synthesis?
A6: Calcination temperature significantly influences particle size, morphology, and magnetic properties. Higher temperatures generally lead to larger particle sizes and enhanced magnetic properties, up to a certain point. [, , ]
Q6: How can the morphology of this compound be controlled during synthesis?
A7: Parameters such as calcination temperature, precursor composition, and the use of additives like salts or surfactants can influence particle morphology. For instance, the addition of NaCl during ultrasonic spray pyrolysis can result in hexagonal plate-shaped particles. []
Q7: Can this compound be prepared in specific morphologies like fibers?
A8: Yes, both microfibers and nanofibers of this compound have been fabricated using methods like sol-gel processing and electrospinning. [, ]
Q8: How does doping with rare-earth elements affect this compound?
A9: Doping with elements like lanthanum (La), samarium (Sm), and cerium (Ce) can influence the magnetic properties of this compound. For example, La-Co substitution can enhance coercivity, while Sm-Mn substitution has been shown to increase both saturation magnetization and coercivity under specific conditions. [, , ]
Q9: What is the impact of Zn substitution on this compound's magnetic properties?
A10: Substitution with zinc (Zn) can increase the residual magnetic induction (Br) of this compound up to a certain concentration, while negatively impacting coercivity. []
Q10: What are the potential applications of this compound?
A11: this compound, due to its magnetic properties, finds applications in various fields, including permanent magnets, magnetic recording media, microwave absorbers, and biomedical applications. [, , , ]
Q11: How is this compound used in microwave absorption?
A12: this compound nanocomposites, particularly when combined with carbon black, show promising microwave absorption properties in the X-band, making them suitable for stealth applications. []
Q12: Can this compound be used in cement-based piezoelectric composites?
A13: Yes, incorporating this compound into cement-based piezoelectric composites can enhance their piezoelectric properties, such as the piezoelectric strain constant (d33) and piezoelectric voltage constant (g33). [, ]
Q13: What is the role of this compound in magnetically separable photocatalysts?
A14: this compound, when loaded onto photocatalysts like TiO2, imparts magnetic properties, allowing for easy separation and recovery of the catalyst after use. These composites have demonstrated efficacy in degrading dyes like methylene blue under UV irradiation. []
Disclaimer and Information on In-Vitro Research Products
Please be aware that all articles and product information presented on BenchChem are intended solely for informational purposes. The products available for purchase on BenchChem are specifically designed for in-vitro studies, which are conducted outside of living organisms. In-vitro studies, derived from the Latin term "in glass," involve experiments performed in controlled laboratory settings using cells or tissues. It is important to note that these products are not categorized as medicines or drugs, and they have not received approval from the FDA for the prevention, treatment, or cure of any medical condition, ailment, or disease. We must emphasize that any form of bodily introduction of these products into humans or animals is strictly prohibited by law. It is essential to adhere to these guidelines to ensure compliance with legal and ethical standards in research and experimentation.