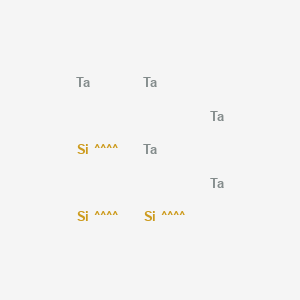
Tantalum silicide
- Click on QUICK INQUIRY to receive a quote from our team of experts.
- With the quality product at a COMPETITIVE price, you can focus more on your research.
Overview
Description
Tantalum silicide is an intermetallic compound composed of tantalum and silicon. It is known for its excellent electrical properties, high melting point, and superior thermal stability. These characteristics make it a valuable material in high-temperature structural applications and microelectronics.
Synthetic Routes and Reaction Conditions:
Combustion Synthesis (Self-Propagating High-Temperature Synthesis): This method involves the exothermic reaction of tantalum and silicon powders.
Arc Melting: Tantalum and silicon are melted together in an arc furnace.
Chemical Vapor Deposition (CVD): Tantalum chloride and silicon precursors are reacted in a vapor phase at high temperatures to deposit this compound on a substrate.
Industrial Production Methods:
Types of Reactions:
Oxidation: this compound can undergo oxidation to form tantalum oxide and silicon dioxide.
Reduction: It can be reduced by hydrogen or other reducing agents to form elemental tantalum and silicon.
Substitution: this compound can participate in substitution reactions where silicon atoms are replaced by other elements.
Common Reagents and Conditions:
Oxidation: Typically occurs at high temperatures in the presence of oxygen.
Reduction: Requires a reducing atmosphere, such as hydrogen gas, at elevated temperatures.
Major Products:
Oxidation: Tantalum oxide (Ta2O5) and silicon dioxide (SiO2).
Reduction: Elemental tantalum (Ta) and silicon (Si).
Scientific Research Applications
Tantalum silicide has a wide range of applications in scientific research and industry:
Microelectronics: Used as a material for gate electrodes, interconnects, and Ohmic contacts in semiconductor devices.
High-Temperature Structural Materials: Its high melting point and thermal stability make it suitable for use in high-temperature environments.
Electrochemical Applications: this compound nanostructures exhibit superior electrochemical capacitance properties, making them useful in energy storage devices.
Optoelectronics: Employed in the fabrication of optical coatings and components.
Mechanism of Action
The mechanism by which tantalum silicide exerts its effects is primarily through its excellent electrical conductivity and thermal stability. The compound forms strong covalent bonds between tantalum and silicon atoms, contributing to its high melting point and resistance to oxidation . The electronic density of states and bonding charge density calculations indicate that the bonding in this compound is mostly covalent in nature .
Comparison with Similar Compounds
- Titanium Silicide (Ti5Si3)
- Molybdenum Silicide (MoSi2)
- Tungsten Silicide (WSi2)
Comparison:
- Titanium Silicide (Ti5Si3): Similar to tantalum silicide, titanium silicide is used in microelectronics for its good electrical properties. this compound has a higher melting point and better thermal stability .
- Molybdenum Silicide (MoSi2): Molybdenum silicide is known for its oxidation resistance and high-temperature strength. This compound, on the other hand, offers superior electrical conductivity .
- Tungsten Silicide (WSi2): Tungsten silicide is used in semiconductor devices for its low resistivity. This compound provides better thermal stability and higher melting point .
This compound stands out due to its unique combination of high melting point, excellent electrical properties, and superior thermal stability, making it a valuable material in various high-temperature and electronic applications.
Biological Activity
Tantalum silicide (TaSi₂) is a compound that has garnered interest in various fields, particularly in materials science and biomedical applications. This article aims to provide a comprehensive overview of its biological activity, highlighting relevant research findings, case studies, and data tables.
Overview of this compound
This compound is primarily known for its applications in microelectronics due to its excellent electrical conductivity and thermal stability. However, its potential biological activity has been explored in recent years, particularly concerning its biocompatibility and antimicrobial properties.
Biocompatibility and Antimicrobial Properties
Research indicates that this compound exhibits promising biocompatibility, making it suitable for biomedical applications. A study focused on the modification of porous tantalum using hydrothermal methods demonstrated that doping with calcium (Ca) and zinc (Zn) can enhance its antibacterial properties. The modified tantalum showed significant antibacterial activity against various pathogens, which is crucial for medical implants and devices .
Table 1: Summary of Biological Activity Studies on this compound
Case Study 1: Antibacterial Properties
In a recent study, researchers synthesized Ca²⁺/Zn²⁺-doped tantalum oxide nanorods from porous tantalum. The biological performance was evaluated through MTT assays and scanning electron microscopy (SEM). The results indicated a marked increase in cell viability and a reduction in bacterial colonization on the surface of the treated materials, suggesting that surface modifications can significantly enhance the antimicrobial efficacy of this compound-based materials .
Case Study 2: Bone Regeneration
Another significant investigation involved the use of additively manufactured porous tantalum implants in a rat femur model. After 12 weeks, substantial bone ingrowth was observed, demonstrating the material's osteoconductive properties. The study highlighted that the mechanical properties of this compound closely resemble those of human bone, facilitating better integration and functionality as an implant material .
Research Findings
Recent findings indicate that this compound can interact positively with physiological solutions, enhancing its bioactivity. For instance, Bio MM and BioRoot RCS, which contain tricalcium silicate and tantalum oxide, showed promising results in terms of flow properties and radiopacity compared to standard materials used in endodontics .
Properties
InChI |
InChI=1S/3Si.5Ta |
Source
|
---|---|---|
Source | PubChem | |
URL | https://pubchem.ncbi.nlm.nih.gov | |
Description | Data deposited in or computed by PubChem | |
InChI Key |
VGQSEFBWEDYXLE-UHFFFAOYSA-N |
Source
|
Source | PubChem | |
URL | https://pubchem.ncbi.nlm.nih.gov | |
Description | Data deposited in or computed by PubChem | |
Canonical SMILES |
[Si].[Si].[Si].[Ta].[Ta].[Ta].[Ta].[Ta] |
Source
|
Source | PubChem | |
URL | https://pubchem.ncbi.nlm.nih.gov | |
Description | Data deposited in or computed by PubChem | |
Molecular Formula |
Si3Ta5 |
Source
|
Source | PubChem | |
URL | https://pubchem.ncbi.nlm.nih.gov | |
Description | Data deposited in or computed by PubChem | |
Molecular Weight |
988.99 g/mol |
Source
|
Source | PubChem | |
URL | https://pubchem.ncbi.nlm.nih.gov | |
Description | Data deposited in or computed by PubChem | |
CAS No. |
12067-56-0 |
Source
|
Record name | Tantalum silicide (Ta5Si3) | |
Source | ChemIDplus | |
URL | https://pubchem.ncbi.nlm.nih.gov/substance/?source=chemidplus&sourceid=0012067560 | |
Description | ChemIDplus is a free, web search system that provides access to the structure and nomenclature authority files used for the identification of chemical substances cited in National Library of Medicine (NLM) databases, including the TOXNET system. | |
Record name | Tantalum silicide (Ta5Si3) | |
Source | EPA Chemicals under the TSCA | |
URL | https://www.epa.gov/chemicals-under-tsca | |
Description | EPA Chemicals under the Toxic Substances Control Act (TSCA) collection contains information on chemicals and their regulations under TSCA, including non-confidential content from the TSCA Chemical Substance Inventory and Chemical Data Reporting. | |
Record name | Pentatantalum trisilicide | |
Source | European Chemicals Agency (ECHA) | |
URL | https://echa.europa.eu/substance-information/-/substanceinfo/100.031.880 | |
Description | The European Chemicals Agency (ECHA) is an agency of the European Union which is the driving force among regulatory authorities in implementing the EU's groundbreaking chemicals legislation for the benefit of human health and the environment as well as for innovation and competitiveness. | |
Explanation | Use of the information, documents and data from the ECHA website is subject to the terms and conditions of this Legal Notice, and subject to other binding limitations provided for under applicable law, the information, documents and data made available on the ECHA website may be reproduced, distributed and/or used, totally or in part, for non-commercial purposes provided that ECHA is acknowledged as the source: "Source: European Chemicals Agency, http://echa.europa.eu/". Such acknowledgement must be included in each copy of the material. ECHA permits and encourages organisations and individuals to create links to the ECHA website under the following cumulative conditions: Links can only be made to webpages that provide a link to the Legal Notice page. | |
Disclaimer and Information on In-Vitro Research Products
Please be aware that all articles and product information presented on BenchChem are intended solely for informational purposes. The products available for purchase on BenchChem are specifically designed for in-vitro studies, which are conducted outside of living organisms. In-vitro studies, derived from the Latin term "in glass," involve experiments performed in controlled laboratory settings using cells or tissues. It is important to note that these products are not categorized as medicines or drugs, and they have not received approval from the FDA for the prevention, treatment, or cure of any medical condition, ailment, or disease. We must emphasize that any form of bodily introduction of these products into humans or animals is strictly prohibited by law. It is essential to adhere to these guidelines to ensure compliance with legal and ethical standards in research and experimentation.