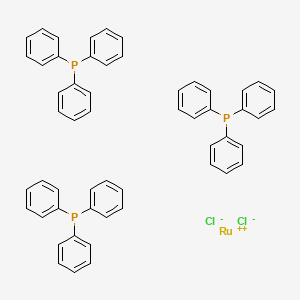
Dichlorotris(triphenylphosphino)ruthenium (II)
Overview
Description
Dichlorotris(triphenylphosphino)ruthenium (II)(II) is a coordination complex of ruthenium. It is a chocolate brown solid that is soluble in organic solvents such as benzene . The compound is used as a precursor to other complexes, including those used in homogeneous catalysis .
Preparation Methods
Synthetic Routes and Reaction Conditions
Dichlorotris(triphenylphosphino)ruthenium (II)(II) is synthesized by reacting ruthenium trichloride trihydrate with a methanolic solution of triphenylphosphine . The reaction can be represented as follows:
2RuCl3(H2O)3+7PPh3→2RuCl2(PPh3)3+2HCl+5H2O+OPPh3
The coordination sphere of Dichlorotris(triphenylphosphino)ruthenium (II)(II) can be viewed as either five-coordinate or octahedral .
Industrial Production Methods
Industrial production methods for Dichlorotris(triphenylphosphino)ruthenium (II)(II) are not extensively documented, but the laboratory synthesis methods are scalable for industrial applications.
Chemical Reactions Analysis
Types of Reactions
Dichlorotris(triphenylphosphino)ruthenium (II)(II) undergoes various types of reactions, including:
Substitution: The triphenylphosphine ligands in Dichlorotris(triphenylphosphino)ruthenium (II)(II) can be readily substituted by other ligands.
Common Reagents and Conditions
Common reagents used in reactions with Dichlorotris(triphenylphosphino)ruthenium (II)(II) include triphenylphosphine, tert-butyl hydroperoxide, and hydrogen in the presence of a base .
Major Products
Major products formed from these reactions include tertiary alcohols, t-butyldioxyamides, and various hydrogenated compounds .
Scientific Research Applications
Dichlorotris(triphenylphosphino)ruthenium (II)(II) has a wide range of scientific research applications:
Chemistry: It is used as a catalyst in organic synthesis, particularly in hydrogenation and oxidation reactions.
Biology: The compound is studied for its potential biological activities and interactions with biomolecules.
Industry: It is utilized in industrial processes for the synthesis of various organic compounds.
Mechanism of Action
The mechanism of action of Dichlorotris(triphenylphosphino)ruthenium (II)(II) involves coordination with substrates through its ruthenium center. The compound can facilitate various catalytic processes by stabilizing transition states and lowering activation energies . The molecular targets and pathways involved depend on the specific reaction and substrate.
Comparison with Similar Compounds
Dichlorotris(triphenylphosphino)ruthenium (II)(II) is unique due to its specific coordination geometry and reactivity. Similar compounds include:
Tris(triphenylphosphine)ruthenium(II) dichloride: Similar in structure but with different reactivity and applications.
Ruthenium tris(triphenylphosphine) dichloride: Another coordination complex with distinct properties.
These compounds share some similarities in their coordination chemistry but differ in their specific applications and reactivity.
Properties
IUPAC Name |
ruthenium(2+);triphenylphosphane;dichloride | |
---|---|---|
Details | Computed by Lexichem TK 2.7.0 (PubChem release 2021.05.07) | |
Source | PubChem | |
URL | https://pubchem.ncbi.nlm.nih.gov | |
Description | Data deposited in or computed by PubChem | |
InChI |
InChI=1S/3C18H15P.2ClH.Ru/c3*1-4-10-16(11-5-1)19(17-12-6-2-7-13-17)18-14-8-3-9-15-18;;;/h3*1-15H;2*1H;/q;;;;;+2/p-2 | |
Details | Computed by InChI 1.0.6 (PubChem release 2021.05.07) | |
Source | PubChem | |
URL | https://pubchem.ncbi.nlm.nih.gov | |
Description | Data deposited in or computed by PubChem | |
InChI Key |
WIWBLJMBLGWSIN-UHFFFAOYSA-L | |
Details | Computed by InChI 1.0.6 (PubChem release 2021.05.07) | |
Source | PubChem | |
URL | https://pubchem.ncbi.nlm.nih.gov | |
Description | Data deposited in or computed by PubChem | |
Canonical SMILES |
C1=CC=C(C=C1)P(C2=CC=CC=C2)C3=CC=CC=C3.C1=CC=C(C=C1)P(C2=CC=CC=C2)C3=CC=CC=C3.C1=CC=C(C=C1)P(C2=CC=CC=C2)C3=CC=CC=C3.[Cl-].[Cl-].[Ru+2] | |
Details | Computed by OEChem 2.3.0 (PubChem release 2021.05.07) | |
Source | PubChem | |
URL | https://pubchem.ncbi.nlm.nih.gov | |
Description | Data deposited in or computed by PubChem | |
Molecular Formula |
C54H45Cl2P3Ru | |
Details | Computed by PubChem 2.1 (PubChem release 2021.05.07) | |
Source | PubChem | |
URL | https://pubchem.ncbi.nlm.nih.gov | |
Description | Data deposited in or computed by PubChem | |
Molecular Weight |
958.8 g/mol | |
Details | Computed by PubChem 2.1 (PubChem release 2021.05.07) | |
Source | PubChem | |
URL | https://pubchem.ncbi.nlm.nih.gov | |
Description | Data deposited in or computed by PubChem | |
CAS No. |
15529-49-4 | |
Record name | Dichlorotris(triphenylphosphine)ruthenium | |
Source | CAS Common Chemistry | |
URL | https://commonchemistry.cas.org/detail?cas_rn=15529-49-4 | |
Description | CAS Common Chemistry is an open community resource for accessing chemical information. Nearly 500,000 chemical substances from CAS REGISTRY cover areas of community interest, including common and frequently regulated chemicals, and those relevant to high school and undergraduate chemistry classes. This chemical information, curated by our expert scientists, is provided in alignment with our mission as a division of the American Chemical Society. | |
Explanation | The data from CAS Common Chemistry is provided under a CC-BY-NC 4.0 license, unless otherwise stated. | |
Record name | Dichlorotris(triphenylphosphine)ruthenium | |
Source | ChemIDplus | |
URL | https://pubchem.ncbi.nlm.nih.gov/substance/?source=chemidplus&sourceid=0015529494 | |
Description | ChemIDplus is a free, web search system that provides access to the structure and nomenclature authority files used for the identification of chemical substances cited in National Library of Medicine (NLM) databases, including the TOXNET system. | |
Record name | Dichlorotris(triphenylphosphine)ruthenium | |
Source | European Chemicals Agency (ECHA) | |
URL | https://echa.europa.eu/substance-information/-/substanceinfo/100.035.957 | |
Description | The European Chemicals Agency (ECHA) is an agency of the European Union which is the driving force among regulatory authorities in implementing the EU's groundbreaking chemicals legislation for the benefit of human health and the environment as well as for innovation and competitiveness. | |
Explanation | Use of the information, documents and data from the ECHA website is subject to the terms and conditions of this Legal Notice, and subject to other binding limitations provided for under applicable law, the information, documents and data made available on the ECHA website may be reproduced, distributed and/or used, totally or in part, for non-commercial purposes provided that ECHA is acknowledged as the source: "Source: European Chemicals Agency, http://echa.europa.eu/". Such acknowledgement must be included in each copy of the material. ECHA permits and encourages organisations and individuals to create links to the ECHA website under the following cumulative conditions: Links can only be made to webpages that provide a link to the Legal Notice page. | |
Retrosynthesis Analysis
AI-Powered Synthesis Planning: Our tool employs the Template_relevance Pistachio, Template_relevance Bkms_metabolic, Template_relevance Pistachio_ringbreaker, Template_relevance Reaxys, Template_relevance Reaxys_biocatalysis model, leveraging a vast database of chemical reactions to predict feasible synthetic routes.
One-Step Synthesis Focus: Specifically designed for one-step synthesis, it provides concise and direct routes for your target compounds, streamlining the synthesis process.
Accurate Predictions: Utilizing the extensive PISTACHIO, BKMS_METABOLIC, PISTACHIO_RINGBREAKER, REAXYS, REAXYS_BIOCATALYSIS database, our tool offers high-accuracy predictions, reflecting the latest in chemical research and data.
Strategy Settings
Precursor scoring | Relevance Heuristic |
---|---|
Min. plausibility | 0.01 |
Model | Template_relevance |
Template Set | Pistachio/Bkms_metabolic/Pistachio_ringbreaker/Reaxys/Reaxys_biocatalysis |
Top-N result to add to graph | 6 |
Feasible Synthetic Routes
Disclaimer and Information on In-Vitro Research Products
Please be aware that all articles and product information presented on BenchChem are intended solely for informational purposes. The products available for purchase on BenchChem are specifically designed for in-vitro studies, which are conducted outside of living organisms. In-vitro studies, derived from the Latin term "in glass," involve experiments performed in controlled laboratory settings using cells or tissues. It is important to note that these products are not categorized as medicines or drugs, and they have not received approval from the FDA for the prevention, treatment, or cure of any medical condition, ailment, or disease. We must emphasize that any form of bodily introduction of these products into humans or animals is strictly prohibited by law. It is essential to adhere to these guidelines to ensure compliance with legal and ethical standards in research and experimentation.