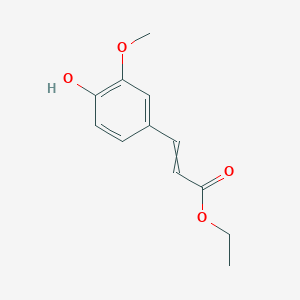
2-Propenoic acid, 3-(4-hydroxy-3-methoxyphenyl)-, ethyl ester
Overview
Description
2-Propenoic acid, 3-(4-hydroxy-3-methoxyphenyl)-, ethyl ester is a natural product found in Tocoyena formosa, Ipomoea nil, and other organisms with data available.
Scientific Research Applications
Cancer Chemoprevention : It has been identified as a potential chemopreventive agent against colon and tongue cancers. Dietary feeding of its derivatives showed promising results in rats (Curini et al., 2006).
Reduction of Aryl-substituted Acids : A study reported the unusual reduction of aryl-substituted 3-hydroxyacrylic acid ester by BH3, resulting in the propenoic acid ethyl ester (Islam et al., 2008).
Biofilm Formation Inhibition : It effectively inhibits biofilm formation by oral pathogens like Porphyromonas gingivalis and Streptococcus mutans, suggesting therapeutic/preventive potential for oral infections (Bodet et al., 2008).
Synthesis of Amyl 4-Hydroxy-3-Methoxycinnamate : Research shows its application in the synthesis of Amyl 4-Hydroxy-3-Methoxycinnamate, a compound of potential industrial and medicinal importance (Xin-jie, 2012).
Spectroscopic and Thermal Studies : Its sodium salt has been analyzed for crystal structure, infrared spectrum, and thermal stability, expanding its potential applications in various fields (Kula et al., 2007).
Anti-Inflammatory Activity : Ester derivatives of 3-(4'-geranyloxy-3'-methoxyphenyl)-2-trans propenoic acid have shown significant topical anti-inflammatory activity, comparable to indomethacin (Epifano et al., 2007).
Synthesis of Ethyl-2-(p-ethoxyphenyl) Propenoate : Its synthesis has been critical in creating ethyl-2-(p-ethoxyphenyl) propenoate, an important intermediate for synthesizing pesticides (Jianquan, 2007).
Synthesis of Naproxen Precursors : It has been used in the synthesis of precursors for Naproxen, a widely used anti-inflammatory drug (Mahmood et al., 2002).
properties
IUPAC Name |
ethyl 3-(4-hydroxy-3-methoxyphenyl)prop-2-enoate | |
---|---|---|
Details | Computed by LexiChem 2.6.6 (PubChem release 2019.06.18) | |
Source | PubChem | |
URL | https://pubchem.ncbi.nlm.nih.gov | |
Description | Data deposited in or computed by PubChem | |
InChI |
InChI=1S/C12H14O4/c1-3-16-12(14)7-5-9-4-6-10(13)11(8-9)15-2/h4-8,13H,3H2,1-2H3 | |
Details | Computed by InChI 1.0.5 (PubChem release 2019.06.18) | |
Source | PubChem | |
URL | https://pubchem.ncbi.nlm.nih.gov | |
Description | Data deposited in or computed by PubChem | |
InChI Key |
ATJVZXXHKSYELS-UHFFFAOYSA-N | |
Details | Computed by InChI 1.0.5 (PubChem release 2019.06.18) | |
Source | PubChem | |
URL | https://pubchem.ncbi.nlm.nih.gov | |
Description | Data deposited in or computed by PubChem | |
Canonical SMILES |
CCOC(=O)C=CC1=CC(=C(C=C1)O)OC | |
Details | Computed by OEChem 2.1.5 (PubChem release 2019.06.18) | |
Source | PubChem | |
URL | https://pubchem.ncbi.nlm.nih.gov | |
Description | Data deposited in or computed by PubChem | |
Molecular Formula |
C12H14O4 | |
Details | Computed by PubChem 2.1 (PubChem release 2019.06.18) | |
Source | PubChem | |
URL | https://pubchem.ncbi.nlm.nih.gov | |
Description | Data deposited in or computed by PubChem | |
DSSTOX Substance ID |
DTXSID001021502 | |
Record name | Ethyl ferulate | |
Source | EPA DSSTox | |
URL | https://comptox.epa.gov/dashboard/DTXSID001021502 | |
Description | DSSTox provides a high quality public chemistry resource for supporting improved predictive toxicology. | |
Molecular Weight |
222.24 g/mol | |
Details | Computed by PubChem 2.1 (PubChem release 2021.05.07) | |
Source | PubChem | |
URL | https://pubchem.ncbi.nlm.nih.gov | |
Description | Data deposited in or computed by PubChem | |
Product Name |
4-Hydroxy-3-methoxycinnamic Acid Ethyl Ester | |
CAS RN |
4046-02-0 | |
Record name | Ethyl ferulate | |
Source | CAS Common Chemistry | |
URL | https://commonchemistry.cas.org/detail?cas_rn=4046-02-0 | |
Description | CAS Common Chemistry is an open community resource for accessing chemical information. Nearly 500,000 chemical substances from CAS REGISTRY cover areas of community interest, including common and frequently regulated chemicals, and those relevant to high school and undergraduate chemistry classes. This chemical information, curated by our expert scientists, is provided in alignment with our mission as a division of the American Chemical Society. | |
Explanation | The data from CAS Common Chemistry is provided under a CC-BY-NC 4.0 license, unless otherwise stated. | |
Record name | Ethyl ferulate | |
Source | EPA DSSTox | |
URL | https://comptox.epa.gov/dashboard/DTXSID001021502 | |
Description | DSSTox provides a high quality public chemistry resource for supporting improved predictive toxicology. | |
Retrosynthesis Analysis
AI-Powered Synthesis Planning: Our tool employs the Template_relevance Pistachio, Template_relevance Bkms_metabolic, Template_relevance Pistachio_ringbreaker, Template_relevance Reaxys, Template_relevance Reaxys_biocatalysis model, leveraging a vast database of chemical reactions to predict feasible synthetic routes.
One-Step Synthesis Focus: Specifically designed for one-step synthesis, it provides concise and direct routes for your target compounds, streamlining the synthesis process.
Accurate Predictions: Utilizing the extensive PISTACHIO, BKMS_METABOLIC, PISTACHIO_RINGBREAKER, REAXYS, REAXYS_BIOCATALYSIS database, our tool offers high-accuracy predictions, reflecting the latest in chemical research and data.
Strategy Settings
Precursor scoring | Relevance Heuristic |
---|---|
Min. plausibility | 0.01 |
Model | Template_relevance |
Template Set | Pistachio/Bkms_metabolic/Pistachio_ringbreaker/Reaxys/Reaxys_biocatalysis |
Top-N result to add to graph | 6 |
Feasible Synthetic Routes
Disclaimer and Information on In-Vitro Research Products
Please be aware that all articles and product information presented on BenchChem are intended solely for informational purposes. The products available for purchase on BenchChem are specifically designed for in-vitro studies, which are conducted outside of living organisms. In-vitro studies, derived from the Latin term "in glass," involve experiments performed in controlled laboratory settings using cells or tissues. It is important to note that these products are not categorized as medicines or drugs, and they have not received approval from the FDA for the prevention, treatment, or cure of any medical condition, ailment, or disease. We must emphasize that any form of bodily introduction of these products into humans or animals is strictly prohibited by law. It is essential to adhere to these guidelines to ensure compliance with legal and ethical standards in research and experimentation.