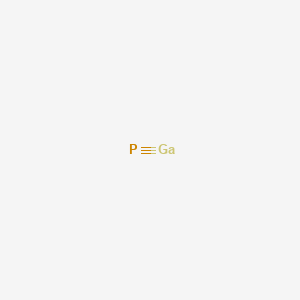
Gallium phosphide
Overview
Description
Gallium phosphide (GaP) is a III-V compound semiconductor with a zinc blende crystal structure (space group F$\overline{4}$3m) and a direct bandgap of 2.26 eV at room temperature . Its high refractive index (~3.3) and nonlinear optical properties make it ideal for photonic applications, including waveguides, resonators, and ultrafast optical modulators . GaP exhibits excellent thermal stability (melting point: 1,467°C) and mechanical robustness, with directional Young’s modulus values ranging from 140–170 GPa depending on crystallographic orientation . Industrially, GaP is synthesized via liquid-encapsulated Czochralsky (LEC) growth or chemical vapor deposition (CVD) to produce high-purity single crystals . Key applications include red, green, and yellow light-emitting diodes (LEDs), high-frequency transistors, and solar cell substrates .
Preparation Methods
Synthetic Routes and Reaction Conditions: Gallium phosphide can be synthesized through several methods, including:
Chemical Vapor Deposition (CVD): This method involves the reaction of gallium trichloride (GaCl3) with phosphine (PH3) at high temperatures. The reaction typically occurs in a hydrogen atmosphere to prevent oxidation.
Liquid Phase Epitaxy (LPE): In this method, gallium and phosphorus are dissolved in a molten solution, and the compound is crystallized on a substrate as the solution cools.
Radio-Frequency Sputter Deposition: This technique involves the deposition of amorphous this compound on a low refractive index glass substrate.
Industrial Production Methods: Industrial production of this compound often involves the use of high-temperature furnaces to grow single crystals from a melt containing gallium and phosphorus. The process must be carefully controlled to prevent the loss of phosphorus as a gas at high temperatures .
Chemical Reactions Analysis
Types of Reactions: Gallium phosphide undergoes various chemical reactions, including:
Oxidation: this compound can be oxidized to form gallium oxide (Ga2O3) and phosphorus pentoxide (P2O5) at high temperatures.
Reduction: It can be reduced back to elemental gallium and phosphorus under specific conditions.
Substitution: this compound can undergo substitution reactions where other elements replace gallium or phosphorus in the lattice structure.
Common Reagents and Conditions:
Oxidizing Agents: Oxygen or air at elevated temperatures.
Reducing Agents: Hydrogen or other reducing gases at high temperatures.
Substituting Agents: Various dopants such as sulfur, silicon, tellurium, and zinc are used to produce n-type and p-type semiconductors.
Major Products:
Oxidation Products: Gallium oxide and phosphorus pentoxide.
Reduction Products: Elemental gallium and phosphorus.
Substitution Products: Doped this compound with altered electrical properties.
Scientific Research Applications
Optoelectronic Devices
High-Efficiency Light Emission
GaP is known for its direct bandgap of approximately 2.26 eV, which allows for efficient light emission. This property makes it ideal for a range of optoelectronic devices:
- Light-Emitting Diodes (LEDs) : GaP is extensively used in the production of red, green, yellow, and orange LEDs due to its transparency to these wavelengths. GaP-based LEDs have demonstrated high efficiency and lower production costs compared to traditional silicon-based devices .
- Laser Diodes : GaP laser diodes operate at higher efficiencies and are used in applications ranging from telecommunications to medical devices.
- Solar Cells : GaP solar cells have achieved efficiencies of up to 20%, making them competitive with silicon solar cells. Their high energy conversion efficiency is attributed to the direct bandgap nature of GaP .
Device Type | Efficiency (%) | Key Application Areas |
---|---|---|
Light-Emitting Diodes (LEDs) | High | Displays, Lighting |
Laser Diodes | Moderate to High | Telecommunications, Medical Devices |
Solar Cells | Up to 20 | Renewable Energy |
Nanophotonics
Metasurfaces and Optical Applications
Recent advancements have highlighted GaP's potential in nanophotonics, particularly in the development of optical metasurfaces. These surfaces are engineered to manipulate light in novel ways:
- Optical Metasurfaces : GaP thin films have been utilized to fabricate metasurfaces with superior optical properties for visible light applications. Research has demonstrated the design and characterization of various metasurface configurations that enhance functionalities such as light manipulation and sensing .
- Nonlinear Optical Effects : GaP exhibits significant nonlinear optical properties, making it suitable for applications such as frequency conversion and optical switching. The material's high refractive index allows for efficient second-harmonic generation and Kerr frequency combs .
Application Type | Description | Notable Properties |
---|---|---|
Optical Metasurfaces | Manipulate light for advanced optical devices | High refractive index, transparency |
Nonlinear Optics | Frequency conversion, optical switching | Strong nonlinear effects |
Biomedical Applications
Cancer Therapy
Gallium compounds, including those derived from GaP, have been investigated for their potential in cancer therapy:
- Antitumor Activity : Research indicates that gallium compounds can inhibit tumor cell proliferation by mimicking iron behavior in biological systems. This mechanism disrupts iron-dependent processes within cancer cells .
- Nanoparticle Delivery Systems : Novel nanoparticle formulations containing gallium have shown enhanced antitumor effects by improving drug delivery efficiency and reducing side effects compared to conventional therapies .
Case Study 1: GaP in LED Technology
A study demonstrated that GaP-based LEDs outperformed traditional silicon-based LEDs in terms of energy efficiency and longevity. The researchers found that by optimizing the doping process with zinc oxide and nitrogen, they could extend the emission wavelength range significantly.
Case Study 2: Metasurfaces for Biomedical Imaging
Researchers developed a GaP metasurface capable of enhancing imaging techniques in biomedical applications. The metasurface was designed to improve resolution and contrast in optical imaging systems, showcasing GaP's potential beyond conventional optics.
Mechanism of Action
The mechanism by which gallium phosphide exerts its effects is primarily through its optical and electronic properties. This compound has a high refractive index and significant nonlinear optical properties, which allow it to confine and manipulate light effectively. Its indirect band gap enables high transmittance except for short wavelengths beyond blue light . The material’s noncentrosymmetric crystal structure contributes to its second-order and third-order nonlinearities, making it suitable for applications such as second-harmonic generation and optical modulation .
Comparison with Similar Compounds
Comparison with Similar III-V Semiconductors
Gallium Arsenide (GaAs)
Property | GaP | GaAs |
---|---|---|
Bandgap (eV) | 2.26 (indirect) | 1.42 (direct) |
Electron Mobility (cm²/Vs) | 110–150 | 8,500–9,200 |
Thermal Conductivity (W/m·K) | 1.1 | 55 |
Primary Applications | LEDs, photonic circuits | RF transistors, solar cells |
- Key Differences : GaAs has superior electron mobility and thermal conductivity, enabling high-speed electronics and photovoltaics, but its narrower bandgap limits use in visible-light LEDs. GaP’s wider bandgap allows emission in the green-yellow spectrum but requires doping (e.g., nitrogen) to improve radiative efficiency .
- Market Share : GaAs dominates 65% of III-V semiconductor production, while GaP accounts for <5% due to niche applications .
Gallium Nitride (GaN)
Property | GaP | GaN |
---|---|---|
Bandgap (eV) | 2.26 | 3.4 |
Breakdown Field (MV/cm) | 0.5–0.7 | 3.3 |
Applications | Low-power LEDs | Blue LEDs, power amplifiers |
- Synthesis : GaN requires heteroepitaxial growth on sapphire or SiC due to lack of native substrates, increasing production costs compared to GaP .
Indium Phosphide (InP)
Property | GaP | InP |
---|---|---|
Bandgap (eV) | 2.26 | 1.34 |
Electron Mobility (cm²/Vs) | 110–150 | 5,400 |
Applications | Visible LEDs | Photodetectors, THz amplifiers |
- Optoelectronic Range : InP covers infrared wavelengths (1,000–1,600 nm), critical for fiber-optic communications, while GaP operates in the visible spectrum .
- Toxicity: InP poses higher carcinogenic risks in occupational settings compared to GaP .
Ternary Alloys: GaAsP and AlGaInP
Property | GaAsP | AlGaInP |
---|---|---|
Bandgap Range (eV) | 1.98–2.26 (adjustable) | 1.81–2.0 |
Applications | Red-orange LEDs | High-brightness LEDs |
- Tunability: GaAsP’s bandgap is modulated by varying the As/P ratio, enabling wavelength-specific LEDs. AlGaInP alloys achieve higher luminous efficiency for displays .
- Efficiency: AlGaInP LEDs achieve 40–60 lm/W, outperforming GaP-based LEDs (10–20 lm/W) due to reduced non-radiative recombination .
Research Findings and Technological Advancements
- Nonlinear Photonics: GaP’s high third-order nonlinear susceptibility ($\chi^{(3)} \approx 10^{-18}$ m²/V²) enables all-optical signal processing in integrated photonic circuits .
- Nanostructured GaP: Amorphous GaP nanocrystals on silica substrates exhibit enhanced surface reactivity and stability, suitable for ultrafast optical switches .
- Thermal Limitations : GaP’s low thermal conductivity restricts use in high-power devices, prompting research into GaP-SiC heterostructures for thermal management .
Biological Activity
Gallium phosphide (GaP) is a compound that has garnered significant attention due to its unique properties and potential applications in various fields, including electronics and biomedicine. This article focuses on the biological activity of GaP, particularly its toxicity and therapeutic potential, supported by recent research findings and case studies.
Overview of this compound
This compound is a III-V semiconductor with a direct bandgap of approximately 2.26 eV, making it suitable for optoelectronic applications. Its high refractive index and transparency in the visible spectrum enhance its utility in photonic devices. However, its biological implications, particularly regarding toxicity and therapeutic applications, are critical areas of study.
Toxicological Studies
Recent studies have highlighted the pulmonary toxicity associated with GaP nanowires. A notable investigation reported that exposure to GaP nanowires induced genotoxicity in bronchoalveolar lavage cells and acute inflammation characterized by eosinophilia. This response was comparable to that induced by multi-walled carbon nanotubes (MWCNTs), indicating potential risks associated with inhalation exposure to GaP .
Key Findings from Toxicological Research
- Genotoxicity : GaP nanowires caused DNA damage in lung cells, leading to an inflammatory response observable within days post-exposure.
- Inflammatory Response : Eosinophilia was significantly noted at 1 and 3 days post-exposure, suggesting an acute allergic or inflammatory reaction .
- Biopersistence : GaP nanowires exhibited a half-life of approximately three months in vivo, raising concerns about long-term accumulation in various organs including the lung, liver, and brain .
Therapeutic Potential
Despite its toxicological concerns, gallium compounds have shown promise in medical applications, particularly in cancer therapy. Gallium acts as an iron mimetic, disrupting iron metabolism in tumor cells, which can inhibit their proliferation.
Applications in Cancer Therapy
Gallium nitrate has been explored for its anticancer properties:
- Mechanism of Action : It interferes with iron-dependent processes in tumor cells, leading to reduced cell viability .
- Clinical Trials : Gallium nitrate has demonstrated efficacy against non-Hodgkin's lymphoma and bladder cancer without the myelosuppressive effects common to many chemotherapeutics .
Case Studies
-
Pulmonary Exposure Study : In a controlled study involving mice, intratracheal instillation of GaP nanowires led to significant eosinophilic inflammation in lung tissues. The study utilized various dosages (2 µg to 18 µg per mouse) and monitored responses at multiple time points (1 day to 3 months post-exposure) .
Dose (µg/mouse) Eosinophil Count (Day 3) 2 Increased 6 Significantly Increased 18 Highest Increase - Gallium Nitrate in Cancer Treatment : A clinical study evaluated the effects of gallium nitrate on patients with advanced cancers. Results indicated a reduction in tumor size and improved patient survival rates without significant toxicity compared to traditional therapies .
Q & A
Basic Research Questions
Q. What are the standard methods for synthesizing high-purity GaP single crystals, and how do parameters like temperature and pressure affect crystal quality?
GaP synthesis typically employs molecular beam epitaxy (MBE) or chemical vapor deposition (CVD) .
- MBE : Requires ultra-high vacuum (10⁻¹⁰ Torr) and precise control of Ga and P fluxes. Substrate temperatures between 500–700°C yield optimal stoichiometry .
- CVD : Uses trimethylgallium (TMGa) and phosphine (PH₃) precursors. Higher pressures (100–300 Torr) reduce defects but may introduce carbon impurities .
Key consideration: Post-growth annealing at 800°C under phosphorus overpressure improves crystallinity by mitigating vacancies .
Q. Which characterization techniques are critical for assessing GaP's structural and electronic properties?
Advanced Research Questions
Q. How do researchers resolve discrepancies in reported bandgap values of GaP under different experimental conditions?
Reported bandgap values range from 2.24–2.34 eV due to:
- Temperature dependence : Bandgap narrows at higher temperatures (e.g., 2.26 eV at 300 K vs. 2.34 eV at 4 K) .
- Doping effects : Tellurium doping introduces shallow states, reducing the effective bandgap by ~10 meV .
Methodological approach: Use pressure-dependent photoluminescence to isolate extrinsic effects. Hydrostatic pressure >5 GPa induces direct-to-indirect bandgap transitions, clarifying intrinsic behavior .
Q. What challenges exist in integrating GaP with silicon-based systems for photonic applications, and what methods improve interfacial stability?
- Lattice mismatch : GaP and Si have a 4% lattice mismatch, causing strain and dislocations.
- Solution : Use graded buffer layers (e.g., SiₓGe₁ₓ) or low-temperature nucleation to minimize interfacial defects .
- Validation : High-resolution TEM and Raman mapping to assess strain distribution .
Q. How does doping with elements like tellurium influence GaP's electronic properties, and what analytical methods validate these changes?
- Tellurium (Te) : Acts as an n-type dopant, introducing shallow donor levels (binding energy ~90 meV) .
- Analytical workflow :
Q. Why do photoluminescence spectra of GaP nanostructures vary significantly across studies, and how can researchers control these variations?
Variations arise from:
- Size effects : Quantum confinement in nanostructures (e.g., nanowires) shifts emission peaks. For 10 nm GaP nanowires, the bandgap increases by ~0.1 eV .
- Surface oxidation : Passivate surfaces with sulfur-containing ligands (e.g., (NH₄)₂S) to suppress non-radiative recombination .
Q. Data Contradiction Analysis
Q. Conflicting reports on GaP’s nonlinear optical coefficients: How to reconcile these differences?
Q. Methodological Recommendations
Properties
IUPAC Name |
gallanylidynephosphane | |
---|---|---|
Source | PubChem | |
URL | https://pubchem.ncbi.nlm.nih.gov | |
Description | Data deposited in or computed by PubChem | |
InChI |
InChI=1S/Ga.P | |
Source | PubChem | |
URL | https://pubchem.ncbi.nlm.nih.gov | |
Description | Data deposited in or computed by PubChem | |
InChI Key |
HZXMRANICFIONG-UHFFFAOYSA-N | |
Source | PubChem | |
URL | https://pubchem.ncbi.nlm.nih.gov | |
Description | Data deposited in or computed by PubChem | |
Canonical SMILES |
P#[Ga] | |
Source | PubChem | |
URL | https://pubchem.ncbi.nlm.nih.gov | |
Description | Data deposited in or computed by PubChem | |
Molecular Formula |
GaP | |
Record name | Gallium phosphide | |
Source | Wikipedia | |
URL | https://en.wikipedia.org/wiki/Gallium_phosphide | |
Description | Chemical information link to Wikipedia. | |
Source | PubChem | |
URL | https://pubchem.ncbi.nlm.nih.gov | |
Description | Data deposited in or computed by PubChem | |
DSSTOX Substance ID |
DTXSID60894184 | |
Record name | Gallium phosphide | |
Source | EPA DSSTox | |
URL | https://comptox.epa.gov/dashboard/DTXSID60894184 | |
Description | DSSTox provides a high quality public chemistry resource for supporting improved predictive toxicology. | |
Molecular Weight |
100.697 g/mol | |
Source | PubChem | |
URL | https://pubchem.ncbi.nlm.nih.gov | |
Description | Data deposited in or computed by PubChem | |
Physical Description |
Amber translucent solid; Greenish-yellow opaque solid if unreacted gallium present; [Merck Index] Pale orange solid; [Alfa Aesar MSDS] | |
Record name | Gallium phosphide | |
Source | Haz-Map, Information on Hazardous Chemicals and Occupational Diseases | |
URL | https://haz-map.com/Agents/20956 | |
Description | Haz-Map® is an occupational health database designed for health and safety professionals and for consumers seeking information about the adverse effects of workplace exposures to chemical and biological agents. | |
Explanation | Copyright (c) 2022 Haz-Map(R). All rights reserved. Unless otherwise indicated, all materials from Haz-Map are copyrighted by Haz-Map(R). No part of these materials, either text or image may be used for any purpose other than for personal use. Therefore, reproduction, modification, storage in a retrieval system or retransmission, in any form or by any means, electronic, mechanical or otherwise, for reasons other than personal use, is strictly prohibited without prior written permission. | |
CAS No. |
12063-98-8 | |
Record name | Gallium phosphide | |
Source | CAS Common Chemistry | |
URL | https://commonchemistry.cas.org/detail?cas_rn=12063-98-8 | |
Description | CAS Common Chemistry is an open community resource for accessing chemical information. Nearly 500,000 chemical substances from CAS REGISTRY cover areas of community interest, including common and frequently regulated chemicals, and those relevant to high school and undergraduate chemistry classes. This chemical information, curated by our expert scientists, is provided in alignment with our mission as a division of the American Chemical Society. | |
Explanation | The data from CAS Common Chemistry is provided under a CC-BY-NC 4.0 license, unless otherwise stated. | |
Record name | Gallium phosphide | |
Source | ChemIDplus | |
URL | https://pubchem.ncbi.nlm.nih.gov/substance/?source=chemidplus&sourceid=0012063988 | |
Description | ChemIDplus is a free, web search system that provides access to the structure and nomenclature authority files used for the identification of chemical substances cited in National Library of Medicine (NLM) databases, including the TOXNET system. | |
Record name | Gallium phosphide (GaP) | |
Source | EPA Chemicals under the TSCA | |
URL | https://www.epa.gov/chemicals-under-tsca | |
Description | EPA Chemicals under the Toxic Substances Control Act (TSCA) collection contains information on chemicals and their regulations under TSCA, including non-confidential content from the TSCA Chemical Substance Inventory and Chemical Data Reporting. | |
Record name | Gallium phosphide | |
Source | EPA DSSTox | |
URL | https://comptox.epa.gov/dashboard/DTXSID60894184 | |
Description | DSSTox provides a high quality public chemistry resource for supporting improved predictive toxicology. | |
Record name | Gallium phosphide | |
Source | European Chemicals Agency (ECHA) | |
URL | https://echa.europa.eu/substance-information/-/substanceinfo/100.031.858 | |
Description | The European Chemicals Agency (ECHA) is an agency of the European Union which is the driving force among regulatory authorities in implementing the EU's groundbreaking chemicals legislation for the benefit of human health and the environment as well as for innovation and competitiveness. | |
Explanation | Use of the information, documents and data from the ECHA website is subject to the terms and conditions of this Legal Notice, and subject to other binding limitations provided for under applicable law, the information, documents and data made available on the ECHA website may be reproduced, distributed and/or used, totally or in part, for non-commercial purposes provided that ECHA is acknowledged as the source: "Source: European Chemicals Agency, http://echa.europa.eu/". Such acknowledgement must be included in each copy of the material. ECHA permits and encourages organisations and individuals to create links to the ECHA website under the following cumulative conditions: Links can only be made to webpages that provide a link to the Legal Notice page. | |
Record name | GALLIUM PHOSPHIDE | |
Source | FDA Global Substance Registration System (GSRS) | |
URL | https://gsrs.ncats.nih.gov/ginas/app/beta/substances/3J421F73DV | |
Description | The FDA Global Substance Registration System (GSRS) enables the efficient and accurate exchange of information on what substances are in regulated products. Instead of relying on names, which vary across regulatory domains, countries, and regions, the GSRS knowledge base makes it possible for substances to be defined by standardized, scientific descriptions. | |
Explanation | Unless otherwise noted, the contents of the FDA website (www.fda.gov), both text and graphics, are not copyrighted. They are in the public domain and may be republished, reprinted and otherwise used freely by anyone without the need to obtain permission from FDA. Credit to the U.S. Food and Drug Administration as the source is appreciated but not required. | |
Disclaimer and Information on In-Vitro Research Products
Please be aware that all articles and product information presented on BenchChem are intended solely for informational purposes. The products available for purchase on BenchChem are specifically designed for in-vitro studies, which are conducted outside of living organisms. In-vitro studies, derived from the Latin term "in glass," involve experiments performed in controlled laboratory settings using cells or tissues. It is important to note that these products are not categorized as medicines or drugs, and they have not received approval from the FDA for the prevention, treatment, or cure of any medical condition, ailment, or disease. We must emphasize that any form of bodily introduction of these products into humans or animals is strictly prohibited by law. It is essential to adhere to these guidelines to ensure compliance with legal and ethical standards in research and experimentation.