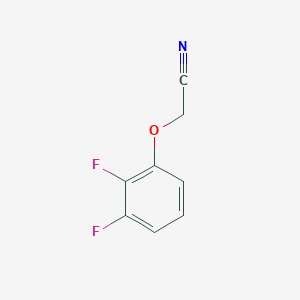
2-(2,3-Difluoro-phenoxy)acetonitrile
Overview
Description
2-(2,3-Difluoro-phenoxy)acetonitrile is a fluorinated aromatic acetonitrile derivative characterized by a phenoxy group substituted with two fluorine atoms at the 2- and 3-positions of the benzene ring, attached to an acetonitrile moiety.
Preparation Methods
Synthetic Routes and Reaction Conditions
The synthesis of 2-(2,3-Difluoro-phenoxy)acetonitrile typically involves the reaction of 2,3-difluorophenol with chloroacetonitrile in the presence of a base such as potassium carbonate. The reaction is carried out in an organic solvent like dimethylformamide (DMF) at elevated temperatures to facilitate the nucleophilic substitution reaction.
Industrial Production Methods
While specific industrial production methods for this compound are not widely documented, the general approach would involve scaling up the laboratory synthesis process. This includes optimizing reaction conditions, such as temperature, solvent, and reaction time, to achieve higher yields and purity suitable for industrial applications.
Chemical Reactions Analysis
Types of Reactions
2-(2,3-Difluoro-phenoxy)acetonitrile can undergo various chemical reactions, including:
Nucleophilic Substitution: The nitrile group can participate in nucleophilic substitution reactions, leading to the formation of amides or other derivatives.
Oxidation and Reduction: The compound can be oxidized or reduced under appropriate conditions to yield different functional groups.
Coupling Reactions: It can participate in coupling reactions such as Suzuki-Miyaura coupling, where it can form carbon-carbon bonds with other aromatic compounds.
Common Reagents and Conditions
Nucleophilic Substitution: Reagents like amines or alcohols in the presence of a base.
Oxidation: Oxidizing agents such as potassium permanganate or hydrogen peroxide.
Reduction: Reducing agents like lithium aluminum hydride or sodium borohydride.
Coupling Reactions: Palladium catalysts and boronic acids or esters.
Major Products Formed
The major products formed from these reactions include amides, alcohols, and various substituted aromatic compounds, depending on the specific reaction conditions and reagents used.
Scientific Research Applications
Organic Synthesis
2-(2,3-Difluoro-phenoxy)acetonitrile serves as a valuable building block in organic synthesis. Its structure allows for various functionalization reactions, enabling the creation of more complex molecules. Recent advancements in synthetic methodologies have highlighted its utility in late-stage functionalization processes, particularly in difluoromethylation reactions that can enhance the biological activity of target compounds .
Research indicates that compounds containing difluorophenoxy groups exhibit significant biological activities, including antimicrobial and anticancer properties. For instance, studies have demonstrated that fluorinated compounds can modulate enzyme activities and receptor interactions, potentially leading to novel therapeutic agents .
Case Study: Antimicrobial Activity
A study evaluating the structure-activity relationship (SAR) of difluorinated compounds revealed that certain derivatives showed promising antibacterial activity against drug-resistant strains of Mycobacterium tuberculosis. The compound's mechanism was linked to the inhibition of specific metabolic pathways, positioning it as a candidate for further drug development .
Medicinal Chemistry
In medicinal chemistry, this compound is being explored as a precursor for drug candidates targeting various diseases. Its unique properties allow for modifications that can enhance pharmacokinetic profiles and target specificity. The compound's ability to interact with biological targets makes it a focus for developing new treatments in oncology and infectious diseases .
Case Study: Drug Development
A recent investigation into the synthesis of radiolabeled tracers for positron emission tomography (PET) highlighted the potential use of this compound in imaging studies. The incorporation of this compound into radiotracers improved their binding affinity and selectivity towards tumor markers, facilitating better imaging outcomes in preclinical models .
Industrial Applications
Beyond laboratory settings, this compound is also utilized in the production of specialty chemicals and agrochemicals. Its unique chemical characteristics make it suitable for formulating products with enhanced efficacy and environmental stability.
Mechanism of Action
The mechanism of action of 2-(2,3-Difluoro-phenoxy)acetonitrile involves its interaction with molecular targets such as enzymes or receptors. The difluorophenoxy group can enhance binding affinity and specificity, while the nitrile group can participate in various chemical reactions, leading to the formation of active metabolites. These interactions can modulate biological pathways and result in specific pharmacological effects.
Comparison with Similar Compounds
Structural Analogues: Fluorinated Phenoxy/Phenylacetonitriles
The table below compares 2-(2,3-Difluoro-phenoxy)acetonitrile with structurally related compounds, emphasizing substituent positions, molecular properties, and key differences:
Key Observations:
Substituent Effects: Electron-Withdrawing vs. Electron-Donating Groups: The 2,3-difluoro-phenoxy group in the target compound creates a strong electron-deficient aromatic system, enhancing reactivity in nucleophilic substitutions. In contrast, methoxy-substituted analogs (e.g., 2-(2-Fluoro-5-methoxyphenyl)acetonitrile) display mixed electronic effects . Positional Isomerism: The 3,5-difluorophenyl analog (symmetric substitution) exhibits higher polarity and thermal stability than asymmetric 2,4-difluorophenyl derivatives .
Molecular Weight and Polarity: The target compound’s molecular weight (~169.12 g/mol) is higher than phenylacetonitrile analogs due to the phenoxy group’s oxygen atom.
Halogenated Phenoxyacetonitriles: Chlorine vs. Fluorine
Comparison with chlorinated analogs reveals significant differences in reactivity and safety:
Key Observations:
Electronegativity and Reactivity : Fluorine’s higher electronegativity (vs. chlorine) in the target compound increases resistance to oxidation and hydrolysis but may enhance susceptibility to nucleophilic attack .
Toxicity : Chlorinated analogs are often more toxic and environmentally persistent, necessitating stricter handling protocols compared to fluorinated derivatives .
Methoxy- and Alkoxy-Substituted Derivatives
Methoxy groups introduce steric and electronic contrasts:
Key Observations:
- Steric Hindrance : The target compound’s smaller substituents (fluoro vs. methoxy/benzyloxy) allow for greater conformational flexibility and accessibility in reactions .
Biological Activity
2-(2,3-Difluoro-phenoxy)acetonitrile is a compound of interest in medicinal chemistry due to its unique structural features and potential biological activities. The presence of fluorine atoms in the phenoxy group can significantly influence the compound's reactivity and interactions with biological targets. This article reviews the biological activity of this compound, focusing on its pharmacological properties, mechanisms of action, and potential therapeutic applications.
Chemical Structure and Properties
The chemical structure of this compound can be represented as follows:
This compound features a phenoxy group substituted with two fluorine atoms at the 2 and 3 positions, which enhances its lipophilicity and may improve its binding affinity to biological targets.
The mechanism of action for this compound primarily involves its interaction with specific enzymes and receptors in biological systems. Research indicates that compounds with similar structures often exhibit activity against cyclooxygenase (COX) enzymes, which are critical in inflammatory processes. For instance, studies have shown that phenoxyacetonitriles can inhibit COX-2, leading to reduced production of pro-inflammatory mediators such as prostaglandins .
Pharmacological Effects
-
Anti-inflammatory Activity :
- Compounds structurally related to this compound have demonstrated significant anti-inflammatory effects. In vivo studies reported reductions in paw thickness and weight in animal models following treatment with similar compounds, suggesting potential use in managing inflammatory diseases .
- Table 1 summarizes the anti-inflammatory effects observed in related compounds:
Compound IC50 (μM) Selectivity Index Compound A 0.06 ± 0.01 111.53 Compound B 0.09 ± 0.01 133.34 Celecoxib 0.05 ± 0.02 298.6 - Antimicrobial Activity :
-
Potential Anticancer Properties :
- Some derivatives have shown cytotoxic effects on cancer cell lines, indicating a possible role in cancer therapy. The specific pathways affected by these compounds require further investigation to establish their efficacy and safety profiles.
Study on Anti-inflammatory Effects
A notable study evaluated a series of phenoxyacetonitriles for their anti-inflammatory activity using a carrageenan-induced paw edema model in rats. The results indicated that certain derivatives significantly reduced edema compared to controls, with a marked decrease in TNF-α levels.
Antimicrobial Evaluation
In another study, the antimicrobial activity of several acetonitrile derivatives was assessed against Staphylococcus aureus and Escherichia coli. The results indicated that some compounds exhibited minimum inhibitory concentrations (MICs) below 10 μg/mL, highlighting their potential as antimicrobial agents .
Safety Profile
Assessing the safety profile of this compound is crucial for its therapeutic application. Toxicological studies have indicated that related compounds do not significantly affect liver enzymes or renal function at therapeutic doses .
Q & A
Basic Research Questions
Q. What are the optimal synthetic routes for preparing 2-(2,3-Difluoro-phenoxy)acetonitrile with high purity?
- Methodology : The compound can be synthesized via nucleophilic substitution between 2,3-difluorophenol and bromoacetonitrile in a polar aprotic solvent (e.g., dimethylformamide or acetonitrile) under basic conditions (e.g., K₂CO₃). Reaction monitoring via TLC or HPLC is recommended to optimize yield and minimize side products like dimerization or incomplete substitution. Purification typically involves column chromatography (silica gel, hexane/ethyl acetate gradient) followed by recrystallization from ethanol .
Q. How can spectroscopic techniques (NMR, IR, MS) distinguish this compound from structurally similar analogs?
- Methodology :
- ¹H/¹⁹F NMR : The fluorine substituents at positions 2 and 3 on the phenyl ring produce distinct splitting patterns. For example, coupling constants (J values) between adjacent fluorine atoms (~15–20 Hz) help confirm substitution patterns.
- IR : A strong nitrile stretch (~2240 cm⁻¹) and aryl C-F vibrations (~1200 cm⁻¹) are diagnostic.
- HRMS : Exact mass analysis (theoretical m/z: 183.03 for C₈H₅F₂NO) confirms molecular ion integrity .
Q. What solvent systems are suitable for recrystallizing this compound?
- Methodology : Acetonitrile-methanol mixtures (7:3 v/v) or ethanol are effective for recrystallization due to moderate solubility and high volatility. Crystallization at low temperatures (0–5°C) improves crystal quality for X-ray analysis. Solvent selection should avoid prolonged exposure to moisture to prevent hydrolysis of the nitrile group .
Q. How does the compound’s stability vary under different storage conditions?
- Methodology : Stability tests in acetonitrile/water mixtures (pH 7.5) show no degradation over 72 hours at 4°C. However, prolonged storage in DMSO or DMF at room temperature may lead to nitrile hydrolysis. Recommend airtight containers with desiccants and storage at –20°C for long-term preservation .
Q. What safety protocols are critical when handling this compound?
- Methodology : Use nitrile gloves, fume hoods, and eye protection. The compound’s acute toxicity (similar to phenylacetonitrile derivatives) requires strict control of inhalation/contact. Waste disposal should follow EPA guidelines for nitrile-containing compounds, using activated carbon filtration for liquid residues .
Advanced Research Questions
Q. How can DFT calculations predict the reactivity of this compound in nucleophilic addition reactions?
- Methodology : Density Functional Theory (B3LYP/6-311+G(d,p)) can model the electron-withdrawing effects of fluorine substituents on the phenyl ring, which polarize the nitrile group and enhance electrophilicity. Frontier molecular orbital analysis (HOMO-LUMO gaps) identifies reactive sites for nucleophilic attack, validated experimentally via kinetic studies in acetonitrile solvent .
Q. What challenges arise in resolving crystal structures of this compound derivatives using SHELX?
- Methodology : Disordered fluorine atoms or nitrile groups complicate refinement. SHELXL-2018’s TWIN and BASF commands improve handling of twinned data. High-resolution datasets (≤1.0 Å) are critical for accurate anisotropic displacement parameter modeling. Example refinement R-factors: <0.05 for well-ordered structures .
Q. How to design an HPLC method for quantifying trace impurities in this compound?
- Methodology : Use a C18 column with a mobile phase of ammonium phosphate buffer (pH 7.5) and acetonitrile-methanol (85:15 v/v). Gradient elution (5–95% organic phase over 20 minutes) resolves impurities like unreacted 2,3-difluorophenol or bromoacetonitrile. UV detection at 210 nm optimizes sensitivity .
Q. What mechanistic insights explain contradictory regioselectivity in cross-coupling reactions involving this compound?
- Methodology : Competing pathways (e.g., Heck vs. Suzuki coupling) depend on palladium catalyst choice. For example, Pd(OAc)₂ with bulky phosphine ligands favors β-hydride elimination in Heck reactions, while Pd(PPh₃)₄ promotes transmetalation in Suzuki systems. Kinetic isotopic effect (KIE) studies and in-situ IR monitor reaction intermediates .
Q. How to mitigate byproduct formation during scale-up synthesis of this compound?
- Methodology :
- Process Optimization : Slow addition of bromoacetonitrile to avoid exothermic side reactions.
- In-line Analytics : PAT tools (e.g., ReactIR) track reaction progression and adjust stoichiometry dynamically.
- Workup : Liquid-liquid extraction (hexane/water) removes polar byproducts before crystallization .
Q. Data Contradiction Analysis
Q. Why do NMR spectra of this compound occasionally show unexpected splitting patterns?
- Analysis : Dynamic fluorine exchange or rotameric equilibria at room temperature may cause signal splitting. Variable-temperature NMR (VT-NMR) between –40°C and 25°C can freeze conformational changes, clarifying true coupling constants. Compare with static DFT-optimized geometries .
Q. How to resolve discrepancies between experimental and computational vibrational spectra?
- Analysis : Anharmonic corrections (e.g., VPT2 method) improve IR frequency predictions. Experimental bands near 1200 cm⁻¹ (C-F stretch) may split due to crystal packing effects, requiring solid-state IR measurements for direct comparison .
Q. Tables
Table 1 : Key Spectroscopic Data for this compound
Table 2 : Solubility Profile in Common Solvents (25°C)
Solvent | Solubility (mg/mL) | Notes |
---|---|---|
Acetonitrile | 120 | Fully miscible |
Ethanol | 45 | Partial crystallization upon cooling |
Water | <1 | Hydrolysis observed at pH >9 |
Properties
IUPAC Name |
2-(2,3-difluorophenoxy)acetonitrile | |
---|---|---|
Details | Computed by LexiChem 2.6.6 (PubChem release 2019.06.18) | |
Source | PubChem | |
URL | https://pubchem.ncbi.nlm.nih.gov | |
Description | Data deposited in or computed by PubChem | |
InChI |
InChI=1S/C8H5F2NO/c9-6-2-1-3-7(8(6)10)12-5-4-11/h1-3H,5H2 | |
Details | Computed by InChI 1.0.5 (PubChem release 2019.06.18) | |
Source | PubChem | |
URL | https://pubchem.ncbi.nlm.nih.gov | |
Description | Data deposited in or computed by PubChem | |
InChI Key |
MVIHBYYWYYFZCZ-UHFFFAOYSA-N | |
Details | Computed by InChI 1.0.5 (PubChem release 2019.06.18) | |
Source | PubChem | |
URL | https://pubchem.ncbi.nlm.nih.gov | |
Description | Data deposited in or computed by PubChem | |
Canonical SMILES |
C1=CC(=C(C(=C1)F)F)OCC#N | |
Details | Computed by OEChem 2.1.5 (PubChem release 2019.06.18) | |
Source | PubChem | |
URL | https://pubchem.ncbi.nlm.nih.gov | |
Description | Data deposited in or computed by PubChem | |
Molecular Formula |
C8H5F2NO | |
Details | Computed by PubChem 2.1 (PubChem release 2019.06.18) | |
Source | PubChem | |
URL | https://pubchem.ncbi.nlm.nih.gov | |
Description | Data deposited in or computed by PubChem | |
Molecular Weight |
169.13 g/mol | |
Details | Computed by PubChem 2.1 (PubChem release 2021.05.07) | |
Source | PubChem | |
URL | https://pubchem.ncbi.nlm.nih.gov | |
Description | Data deposited in or computed by PubChem | |
Disclaimer and Information on In-Vitro Research Products
Please be aware that all articles and product information presented on BenchChem are intended solely for informational purposes. The products available for purchase on BenchChem are specifically designed for in-vitro studies, which are conducted outside of living organisms. In-vitro studies, derived from the Latin term "in glass," involve experiments performed in controlled laboratory settings using cells or tissues. It is important to note that these products are not categorized as medicines or drugs, and they have not received approval from the FDA for the prevention, treatment, or cure of any medical condition, ailment, or disease. We must emphasize that any form of bodily introduction of these products into humans or animals is strictly prohibited by law. It is essential to adhere to these guidelines to ensure compliance with legal and ethical standards in research and experimentation.