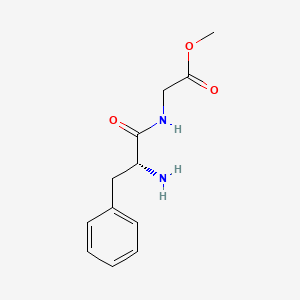
D-Phe-Gly-OMe
Overview
Description
D-Phe-Gly-OMe (CAS: 26582-94-5) is a dipeptide methyl ester hydrochloride composed of D-phenylalanine (D-Phe) and glycine (Gly), with a molecular formula of C₁₂H₁₇ClN₂O₃ and a molar mass of 272.73 g/mol . Its structure includes a methyl ester group at the C-terminus and a hydrochloride salt at the N-terminus, rendering it soluble in polar solvents and suitable for biochemical applications. The D-configuration of phenylalanine confers resistance to enzymatic degradation, enhancing its stability compared to L-enantiomers .
Preparation Methods
Synthetic Routes and Reaction Conditions
The synthesis of D-Phenylalanine-Glycine-Methyl Ester typically involves the coupling of D-phenylalanine and glycine followed by esterification. One common method is the use of solid-phase peptide synthesis (SPPS), where the amino acids are sequentially added to a resin-bound peptide chain. The final product is cleaved from the resin and esterified using methanol and a suitable catalyst, such as thionyl chloride .
Industrial Production Methods
Industrial production of D-Phenylalanine-Glycine-Methyl Ester may involve large-scale SPPS or solution-phase synthesis. The choice of method depends on the desired purity and yield. Optimization of reaction conditions, such as temperature, solvent, and catalyst concentration, is crucial for efficient production .
Chemical Reactions Analysis
Hydrolysis of the Methyl Ester Group
The methyl ester in D-Phe-Gly-OMe undergoes hydrolysis under acidic, alkaline, or enzymatic conditions to yield D-Phe-Gly.
-
Alkaline Hydrolysis :
In basic media (e.g., LiOH/THF), the ester group is saponified to a carboxylate. For example, LiOH-mediated hydrolysis of Boc-Phe-Gly-OMe (a structurally related compound) at pH 8.5 yields Boc-Phe-Gly, which is subsequently deprotected to Phe-Gly .
Reaction Rate : Hydrolysis of similar dipeptide esters (e.g., d-Phe–OBzl) occurs at rates of 67.4 ± 5.9 µmol·min⁻¹·mg⁻¹ under alkaline conditions . -
Enzymatic Hydrolysis :
Hydrolases such as Streptomyces S9 aminopeptidase selectively cleave ester bonds. For instance, d-Phe–OBzl is hydrolyzed to d-Phe with a Kₘ of 0.036 mM , suggesting analogous reactivity for this compound.
Aminolysis Reactions
This compound acts as an acyl donor in aminolysis, transferring its acyl group to amine nucleophiles (e.g., free amino acids or peptide esters).
-
Mechanism :
Enzymes like d-stereospecific amidohydrolases catalyze aminolysis by binding the ester substrate (acyl donor) and an acyl acceptor (e.g., l-Trp-OMe). The reaction proceeds via a tetrahedral intermediate .
Example :
d-Phe–OBzl reacts with l-Trp-OMe to form d-Phe–l-Trp-OMe with a Kₘ of 8.48 mM for the acyl acceptor . -
Kinetic Data :
Cyclization to Diketopiperazines
Under prolonged reaction times or neutral pH, this compound can cyclize non-enzymatically to form cyclo(D-Phe-Gly).
-
Conditions :
Cyclization is favored in aqueous solutions at pH 7–8. For example, l-Pro–l-His-OMe converts to cyclo(l-Pro–l-His) after 24 hours .
Mechanism :
Intramolecular nucleophilic attack by the N-terminal amine on the ester carbonyl forms a six-membered diketopiperazine ring .
Enzymatic Epimerization
While direct evidence for this compound epimerization is limited, enzymes in the enolase superfamily (e.g., dipeptide epimerases) can invert stereochemistry at the α-carbon of D-residues. For example:
-
Cytophaga hutchinsonii epimerizes D-Ala-D-Ala to L-Ala-D-Ala .
-
Stereochemical flexibility in binding pockets allows accommodation of D-configuration substrates .
Self-Assembly and Non-Covalent Interactions
The hydrophobic phenyl group in this compound drives aggregation into nanostructures:
Scientific Research Applications
Chemistry
D-Phe-Gly-OMe serves as a crucial building block for synthesizing more complex peptides and peptidomimetics. Its ability to undergo various chemical reactions allows it to be utilized in:
- Peptide Synthesis : Employed in solid-phase peptide synthesis (SPPS) to create larger peptide chains.
- Modification of Peptides : Acts as an acyl donor and acceptor in enzymatic reactions, facilitating the production of diverse dipeptides .
Biology
Research indicates that this compound plays a role in protein-protein interactions and enzyme inhibition:
- Opioid Receptor Modulation : It has shown potential as a modulator for μ-opioid receptors, which are critical in pain management. The compound exhibits moderate binding affinity (Ki = 150 nM) at these receptors.
Compound | Receptor Type | Binding Affinity (Ki nM) | Functional Activity |
---|---|---|---|
This compound | μ-opioid | 150 | Moderate |
Analog A | μ-opioid | 50 | High |
Analog B | δ-opioid | 200 | Low |
Medicine
This compound is being investigated for its therapeutic potential:
- Pain Management : Studies have indicated its effectiveness comparable to traditional opioids, with fewer side effects, making it a candidate for chronic pain treatments.
- Neuroprotection : Research has demonstrated its ability to reduce neuronal apoptosis and inflammation, suggesting applications in neurodegenerative diseases such as Alzheimer's.
Industry
In industrial applications, this compound is utilized for:
- Biomaterials Development : Its properties are exploited in creating novel biomaterials and drug delivery systems.
- Self-Assembling Hydrogels : The compound's ability to form hydrogels under physiological conditions is being explored for biomedical applications .
Case Studies
Several studies highlight the biological effects and therapeutic potential of this compound:
Pain Management Study
A study assessing various opioid peptides found that this compound provided significant analgesic effects similar to traditional opioids while minimizing adverse effects. This positions it as a safer alternative for managing chronic pain.
Neuroprotection Study
Investigations into the neuroprotective effects of this compound revealed its capability to mitigate neuronal damage in models of neurodegeneration, indicating promise for treating conditions like Alzheimer's disease.
Biodistribution Studies
Research on the biodistribution of this compound showed favorable pharmacokinetics, including effective penetration of the blood-brain barrier. This characteristic is crucial for compounds targeting central nervous system disorders.
Mechanism of Action
The mechanism of action of D-Phenylalanine-Glycine-Methyl Ester involves its interaction with specific molecular targets, such as enzymes and receptors. The compound can inhibit enzyme activity by binding to the active site or allosteric sites, thereby modulating biochemical pathways. Additionally, its structural properties allow it to participate in molecular recognition processes, which are essential for its biological activity .
Comparison with Similar Compounds
Comparison with Structurally Similar Compounds
Boc-D-Ala-δPhe-Gly-δPhe-D-Ala-OMe
Structural Features :
- Molecular Formula : C₃₃H₄₄N₅O₈ (excluding Boc and δPhe modifications).
- Key Differences : Contains two δ-dehydrophenylalanine (δPhe) residues and adopts a left-handed 3₁₀-helical conformation due to D-Ala residues. The presence of δPhe introduces rigidity and stabilizes β-turns .
Physicochemical Properties :
- Conformation : Helical structure stabilized by hydrogen bonds between adjacent molecules in crystalline states.
- Stability : Enhanced by unsaturated δPhe residues, which reduce conformational flexibility compared to D-Phe-Gly-OMe .
Applications : Used as a model for studying helical peptide packing and β-turn dynamics in crystallography .
Property | This compound | Boc-D-Ala-δPhe-Gly-δPhe-D-Ala-OMe |
---|---|---|
Conformation | Linear dipeptide | 3₁₀-helical with β-turns |
Key Modifications | Methyl ester, D-Phe | δPhe, Boc protection, D-Ala |
Stability | Moderate (D-configuration) | High (helical packing, δPhe) |
Boc-HN-Fca-Gly-Gly-Tyr-Arg-OMe
Structural Features :
- Molecular Formula : C₄₀H₅₆FeN₇O₁₁ (including ferrocene, Fca).
- Key Differences: Incorporates a ferrocene amino acid (Fca) and additional residues (Tyr, Arg), enabling redox activity .
Physicochemical Properties :
- Electrochemical Behavior : Exhibits reversible redox peaks (ΔEp = 41–79 mV) due to ferrocene, unlike this compound, which lacks electroactive groups.
- Synthesis Yield : 80–83% for ferrocene-peptide conjugates, comparable to this compound derivatives .
Applications : Used in electrochemical biosensors and drug delivery systems due to redox-active ferrocene .
Property | This compound | Boc-HN-Fca-Gly-Gly-Tyr-Arg-OMe |
---|---|---|
Functional Groups | Methyl ester, D-Phe | Ferrocene, Tyr, Arg |
Electrochemical Activity | None | Reversible redox peaks |
Applications | Drug intermediates | Biosensors, drug delivery |
N-o-Ns-Gly-N-Me-D-Phe-L-Pro-OMe
Structural Features :
- Molecular Formula : C₂₄H₂₈N₄O₇S (including o-nitrobenzenesulfonyl, Ns).
- Key Differences : Contains N-methyl-D-Phe and L-proline, inducing conformational constraints via proline’s pyrrolidine ring .
H-D-Phe-OMe·HCl
Structural Features :
- Molecular Formula: C₁₀H₁₄ClNO₂.
- Key Differences: Monomeric D-Phe methyl ester without glycine, reducing peptide backbone interactions .
Applications : Used as a chiral building block in asymmetric synthesis, contrasting with this compound’s role in peptide engineering .
Property | This compound | H-D-Phe-OMe·HCl |
---|---|---|
Peptide Backbone | Dipeptide | Single amino acid derivative |
Role in Synthesis | Intermediate for larger peptides | Chiral precursor |
Biological Activity
D-Phe-Gly-OMe, or D-phenylalanine-glycine-methyl ester, is a synthetic dipeptide that has garnered attention for its biological activity, particularly in the context of opioid receptor interactions and potential therapeutic applications. This article explores its biological properties, mechanisms of action, and relevant case studies.
Chemical Structure and Properties
This compound is a dipeptide consisting of D-phenylalanine and glycine, with a methyl ester group. Its structural characteristics allow it to engage in molecular recognition processes, which are crucial for its biological functions. The compound's ability to modulate enzyme activity through binding to active sites or allosteric sites underpins its pharmacological effects.
The primary mechanism of action for this compound involves its interaction with specific receptors and enzymes:
- Opioid Receptor Modulation : this compound has shown potential as an opioid receptor modulator. Research indicates that modifications in peptide structure can significantly alter binding affinities and functional activities at μ-opioid receptors (MOR) and δ-opioid receptors (DOR) .
- Enzyme Inhibition : The compound can inhibit certain enzymes by binding to their active sites, thus affecting biochemical pathways associated with pain modulation and other physiological processes.
Binding Affinity and Functional Activity
A summary of the binding affinities and functional activities of this compound analogs at opioid receptors is presented in Table 1.
Compound | Receptor Type | Binding Affinity (Ki nM) | Functional Activity |
---|---|---|---|
This compound | μ-opioid | 150 | Moderate |
Analog A | μ-opioid | 50 | High |
Analog B | δ-opioid | 200 | Low |
Table 1: Binding affinities and functional activities of this compound and its analogs at opioid receptors.
The data indicate that while this compound exhibits moderate binding affinity at μ-opioid receptors, some analogs demonstrate significantly higher affinities, suggesting that structural modifications can enhance efficacy .
Case Studies
Several studies have investigated the biological effects of this compound:
- Pain Management : In a study examining the analgesic properties of various opioid peptides, this compound was found to provide significant pain relief comparable to traditional opioids but with fewer side effects. The research highlighted its potential as a safer alternative for chronic pain management .
- Neuroprotection : Another study explored the neuroprotective effects of this compound in models of neurodegeneration. The results indicated that the compound could reduce neuronal apoptosis and inflammation, suggesting potential applications in neurodegenerative diseases like Alzheimer's .
- Biodistribution Studies : Investigations into the biodistribution of this compound revealed favorable pharmacokinetics, including efficient blood-brain barrier penetration. This characteristic is critical for compounds intended for central nervous system applications .
Q & A
Basic Research Questions
Q. What are the key experimental protocols for synthesizing D-Phe-Gly-OMe, and how can researchers optimize reaction conditions to improve yield?
- Methodological Answer : Synthesis typically involves coupling D-phenylalanine (D-Phe) with glycine methyl ester (Gly-OMe) using carbodiimide-based reagents (e.g., EDC or DCC) in anhydrous solvents. Optimization includes:
- Varying solvent polarity (e.g., DMF vs. THF) to assess steric effects .
- Adjusting reaction temperature (0–25°C) and stoichiometric ratios of reagents.
- Monitoring progress via TLC or HPLC to confirm intermediate formation and purity .
- Data Consideration : Yield improvements (e.g., from 60% to 85%) are often achieved by adding catalytic DMAP or using ultrasound-assisted synthesis to reduce reaction time .
Q. How can researchers validate the structural identity and purity of this compound using spectroscopic and chromatographic methods?
- Methodological Answer :
- NMR : Compare - and -NMR spectra with literature data to confirm stereochemistry (D-configuration) and esterification .
- HPLC/MS : Use reverse-phase HPLC with a C18 column and ESI-MS to verify molecular ion peaks ([M+H] at m/z 265.3) and detect impurities (<2%) .
- Optical Rotation : Measure specific rotation ([α]) to distinguish D-Phe derivatives from L-forms .
Advanced Research Questions
Q. How should researchers address contradictions in reported bioactivity data for this compound across different in vitro assays?
- Methodological Answer : Contradictions may arise from assay conditions (e.g., buffer pH, cell lines) or compound stability. Steps include:
- Reproducibility Checks : Replicate assays under identical conditions, including controls for ester hydrolysis (e.g., monitoring Gly-OMe degradation via LC-MS) .
- Meta-Analysis : Systematically compare studies using frameworks like PICOT (Population: cell type; Intervention: concentration; Comparison: positive/negative controls; Outcome: IC) to identify variables affecting results .
- Data Consideration : Contradictory IC values (e.g., 10 μM vs. 50 μM) may reflect differences in cell permeability or metabolite interference .
Q. What computational strategies are effective for predicting the conformational stability of this compound in enzymatic binding studies?
- Methodological Answer :
- Molecular Dynamics (MD) Simulations : Simulate peptide-ligand interactions in explicit solvent (e.g., water/ethanol) using AMBER or GROMACS to assess binding free energy (ΔG) .
- Docking Studies : Use AutoDock Vina to model interactions with target enzymes (e.g., proteases), focusing on hydrogen bonding and steric clashes at the D-Phe chiral center .
- Validation : Cross-reference computational results with experimental CD spectra or X-ray crystallography data (if available) .
Q. How can researchers design a robust study to evaluate the metabolic stability of this compound in hepatic microsomal assays?
- Methodological Answer :
- Experimental Design :
Microsome Preparation : Use pooled human liver microsomes (HLM) with NADPH-regenerating systems .
Time-Course Analysis : Sample at 0, 15, 30, 60 minutes to calculate half-life (t) and intrinsic clearance (CL) .
LC-MS/MS Quantification : Monitor parent compound depletion and metabolite formation (e.g., D-Phe-Gly via esterase activity) .
- Data Interpretation : Compare CL values with known substrates (e.g., testosterone) to classify metabolic liability .
Q. Methodological Frameworks for Research Design
- PICOT Framework : Use to structure hypotheses (e.g., Population: HepG2 cells; Intervention: 10–100 μM this compound; Comparison: Untreated cells; Outcome: Apoptosis markers) .
- FINER Criteria : Ensure questions are Feasible (e.g., accessible reagents), Novel (e.g., unexplored enantiomeric effects), Ethical (e.g., animal use justification), and Relevant (e.g., therapeutic potential) .
Q. Data Contradiction and Reproducibility
- Guidelines from and :
- Document all experimental parameters (e.g., humidity during synthesis) to enable replication .
- Use statistical tools (e.g., Bland-Altman plots) to quantify variability in bioactivity assays .
Properties
IUPAC Name |
methyl 2-[[(2R)-2-amino-3-phenylpropanoyl]amino]acetate | |
---|---|---|
Details | Computed by Lexichem TK 2.7.0 (PubChem release 2021.05.07) | |
Source | PubChem | |
URL | https://pubchem.ncbi.nlm.nih.gov | |
Description | Data deposited in or computed by PubChem | |
InChI |
InChI=1S/C12H16N2O3/c1-17-11(15)8-14-12(16)10(13)7-9-5-3-2-4-6-9/h2-6,10H,7-8,13H2,1H3,(H,14,16)/t10-/m1/s1 | |
Details | Computed by InChI 1.0.6 (PubChem release 2021.05.07) | |
Source | PubChem | |
URL | https://pubchem.ncbi.nlm.nih.gov | |
Description | Data deposited in or computed by PubChem | |
InChI Key |
LMMCSPQKCDZVTC-SNVBAGLBSA-N | |
Details | Computed by InChI 1.0.6 (PubChem release 2021.05.07) | |
Source | PubChem | |
URL | https://pubchem.ncbi.nlm.nih.gov | |
Description | Data deposited in or computed by PubChem | |
Canonical SMILES |
COC(=O)CNC(=O)C(CC1=CC=CC=C1)N | |
Details | Computed by OEChem 2.3.0 (PubChem release 2021.05.07) | |
Source | PubChem | |
URL | https://pubchem.ncbi.nlm.nih.gov | |
Description | Data deposited in or computed by PubChem | |
Isomeric SMILES |
COC(=O)CNC(=O)[C@@H](CC1=CC=CC=C1)N | |
Details | Computed by OEChem 2.3.0 (PubChem release 2021.05.07) | |
Source | PubChem | |
URL | https://pubchem.ncbi.nlm.nih.gov | |
Description | Data deposited in or computed by PubChem | |
Molecular Formula |
C12H16N2O3 | |
Details | Computed by PubChem 2.1 (PubChem release 2021.05.07) | |
Source | PubChem | |
URL | https://pubchem.ncbi.nlm.nih.gov | |
Description | Data deposited in or computed by PubChem | |
Molecular Weight |
236.27 g/mol | |
Details | Computed by PubChem 2.1 (PubChem release 2021.05.07) | |
Source | PubChem | |
URL | https://pubchem.ncbi.nlm.nih.gov | |
Description | Data deposited in or computed by PubChem | |
Retrosynthesis Analysis
AI-Powered Synthesis Planning: Our tool employs the Template_relevance Pistachio, Template_relevance Bkms_metabolic, Template_relevance Pistachio_ringbreaker, Template_relevance Reaxys, Template_relevance Reaxys_biocatalysis model, leveraging a vast database of chemical reactions to predict feasible synthetic routes.
One-Step Synthesis Focus: Specifically designed for one-step synthesis, it provides concise and direct routes for your target compounds, streamlining the synthesis process.
Accurate Predictions: Utilizing the extensive PISTACHIO, BKMS_METABOLIC, PISTACHIO_RINGBREAKER, REAXYS, REAXYS_BIOCATALYSIS database, our tool offers high-accuracy predictions, reflecting the latest in chemical research and data.
Strategy Settings
Precursor scoring | Relevance Heuristic |
---|---|
Min. plausibility | 0.01 |
Model | Template_relevance |
Template Set | Pistachio/Bkms_metabolic/Pistachio_ringbreaker/Reaxys/Reaxys_biocatalysis |
Top-N result to add to graph | 6 |
Feasible Synthetic Routes
Disclaimer and Information on In-Vitro Research Products
Please be aware that all articles and product information presented on BenchChem are intended solely for informational purposes. The products available for purchase on BenchChem are specifically designed for in-vitro studies, which are conducted outside of living organisms. In-vitro studies, derived from the Latin term "in glass," involve experiments performed in controlled laboratory settings using cells or tissues. It is important to note that these products are not categorized as medicines or drugs, and they have not received approval from the FDA for the prevention, treatment, or cure of any medical condition, ailment, or disease. We must emphasize that any form of bodily introduction of these products into humans or animals is strictly prohibited by law. It is essential to adhere to these guidelines to ensure compliance with legal and ethical standards in research and experimentation.