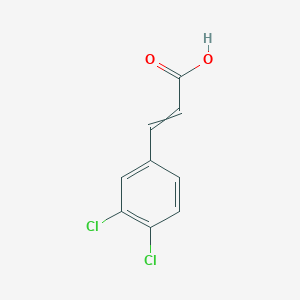
3-(3,4-Dichlorophenyl)prop-2-enoic acid
Overview
Description
3-(3,4-Dichlorophenyl)prop-2-enoic acid is an organic compound with the molecular formula C9H6Cl2O2 It is characterized by the presence of a dichlorophenyl group attached to a prop-2-enoic acid moiety
Preparation Methods
Synthetic Routes and Reaction Conditions
The synthesis of 3-(3,4-Dichlorophenyl)prop-2-enoic acid typically involves the reaction of 3,4-dichlorobenzaldehyde with malonic acid in the presence of a base, followed by decarboxylation. The reaction conditions often include the use of a solvent such as ethanol and a base like sodium ethoxide. The mixture is refluxed, and the product is isolated through filtration and recrystallization .
Industrial Production Methods
Industrial production methods for this compound may involve similar synthetic routes but on a larger scale. The use of continuous flow reactors and automated systems can enhance the efficiency and yield of the production process.
Chemical Reactions Analysis
Types of Reactions
3-(3,4-Dichlorophenyl)prop-2-enoic acid can undergo various chemical reactions, including:
Oxidation: The compound can be oxidized to form corresponding carboxylic acids or ketones.
Reduction: Reduction reactions can convert the compound into alcohols or alkanes.
Substitution: Electrophilic substitution reactions can introduce different functional groups into the aromatic ring.
Common Reagents and Conditions
Oxidation: Common oxidizing agents include potassium permanganate (KMnO4) and chromium trioxide (CrO3).
Reduction: Reducing agents such as lithium aluminum hydride (LiAlH4) and sodium borohydride (NaBH4) are frequently used.
Substitution: Reagents like halogens (e.g., bromine) and nitrating agents (e.g., nitric acid) are used under controlled conditions.
Major Products
The major products formed from these reactions depend on the specific reagents and conditions used. For example, oxidation can yield carboxylic acids, while reduction can produce alcohols.
Scientific Research Applications
3-(3,4-Dichlorophenyl)prop-2-enoic acid has several scientific research applications:
Chemistry: It is used as a building block in the synthesis of more complex organic molecules.
Medicine: Research has explored its potential as an anti-inflammatory and anticancer agent.
Industry: It is used in the production of various pharmaceuticals and agrochemicals.
Mechanism of Action
The mechanism of action of 3-(3,4-Dichlorophenyl)prop-2-enoic acid involves its interaction with molecular targets such as enzymes and receptors. The compound can modulate the activity of these targets, leading to various biological effects. For example, its antioxidant activity is attributed to its ability to scavenge ROS and upregulate the expression of protective proteins like HO-1 and GCLC .
Comparison with Similar Compounds
Similar Compounds
3-(2,4-Dichlorophenyl)propionic acid: Similar in structure but with different substitution patterns on the aromatic ring.
3-(3,4-Dichlorophenyl)propionic acid: A related compound with a saturated propionic acid moiety instead of the unsaturated prop-2-enoic acid.
Uniqueness
3-(3,4-Dichlorophenyl)prop-2-enoic acid is unique due to its specific substitution pattern and the presence of the prop-2-enoic acid moiety. This structure imparts distinct chemical and biological properties, making it valuable for various applications.
Properties
IUPAC Name |
3-(3,4-dichlorophenyl)prop-2-enoic acid | |
---|---|---|
Details | Computed by LexiChem 2.6.6 (PubChem release 2019.06.18) | |
Source | PubChem | |
URL | https://pubchem.ncbi.nlm.nih.gov | |
Description | Data deposited in or computed by PubChem | |
InChI |
InChI=1S/C9H6Cl2O2/c10-7-3-1-6(5-8(7)11)2-4-9(12)13/h1-5H,(H,12,13) | |
Details | Computed by InChI 1.0.5 (PubChem release 2019.06.18) | |
Source | PubChem | |
URL | https://pubchem.ncbi.nlm.nih.gov | |
Description | Data deposited in or computed by PubChem | |
InChI Key |
RRLUFPHCTSFKNR-UHFFFAOYSA-N | |
Details | Computed by InChI 1.0.5 (PubChem release 2019.06.18) | |
Source | PubChem | |
URL | https://pubchem.ncbi.nlm.nih.gov | |
Description | Data deposited in or computed by PubChem | |
Canonical SMILES |
C1=CC(=C(C=C1C=CC(=O)O)Cl)Cl | |
Details | Computed by OEChem 2.1.5 (PubChem release 2019.06.18) | |
Source | PubChem | |
URL | https://pubchem.ncbi.nlm.nih.gov | |
Description | Data deposited in or computed by PubChem | |
Molecular Formula |
C9H6Cl2O2 | |
Details | Computed by PubChem 2.1 (PubChem release 2019.06.18) | |
Source | PubChem | |
URL | https://pubchem.ncbi.nlm.nih.gov | |
Description | Data deposited in or computed by PubChem | |
DSSTOX Substance ID |
DTXSID101311887 | |
Record name | 3,4-Dichlorocinnamic acid | |
Source | EPA DSSTox | |
URL | https://comptox.epa.gov/dashboard/DTXSID101311887 | |
Description | DSSTox provides a high quality public chemistry resource for supporting improved predictive toxicology. | |
Molecular Weight |
217.05 g/mol | |
Details | Computed by PubChem 2.1 (PubChem release 2021.05.07) | |
Source | PubChem | |
URL | https://pubchem.ncbi.nlm.nih.gov | |
Description | Data deposited in or computed by PubChem | |
CAS No. |
1202-39-7 | |
Record name | 3,4-Dichlorocinnamic acid | |
Source | CAS Common Chemistry | |
URL | https://commonchemistry.cas.org/detail?cas_rn=1202-39-7 | |
Description | CAS Common Chemistry is an open community resource for accessing chemical information. Nearly 500,000 chemical substances from CAS REGISTRY cover areas of community interest, including common and frequently regulated chemicals, and those relevant to high school and undergraduate chemistry classes. This chemical information, curated by our expert scientists, is provided in alignment with our mission as a division of the American Chemical Society. | |
Explanation | The data from CAS Common Chemistry is provided under a CC-BY-NC 4.0 license, unless otherwise stated. | |
Record name | 3,4-Dichlorocinnamic acid | |
Source | EPA DSSTox | |
URL | https://comptox.epa.gov/dashboard/DTXSID101311887 | |
Description | DSSTox provides a high quality public chemistry resource for supporting improved predictive toxicology. | |
Disclaimer and Information on In-Vitro Research Products
Please be aware that all articles and product information presented on BenchChem are intended solely for informational purposes. The products available for purchase on BenchChem are specifically designed for in-vitro studies, which are conducted outside of living organisms. In-vitro studies, derived from the Latin term "in glass," involve experiments performed in controlled laboratory settings using cells or tissues. It is important to note that these products are not categorized as medicines or drugs, and they have not received approval from the FDA for the prevention, treatment, or cure of any medical condition, ailment, or disease. We must emphasize that any form of bodily introduction of these products into humans or animals is strictly prohibited by law. It is essential to adhere to these guidelines to ensure compliance with legal and ethical standards in research and experimentation.