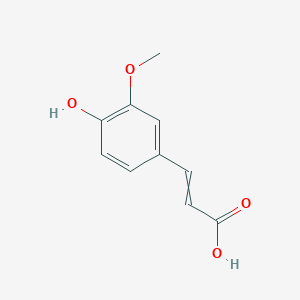
3-(4-Hydroxy-3-methoxyphenyl)prop-2-enoic acid
Overview
Description
3-(4-hydroxy-3-methoxyphenyl)prop-2-enoic acid is a hydroxycinnamic acid.
3-(4-Hydroxy-3-methoxyphenyl)prop-2-enoic acid is a natural product found in Chaerophyllum hirsutum, Astragalus onobrychis, and other organisms with data available.
Scientific Research Applications
Structural and Spectroscopic Analysis
- The compound has been characterized through X-ray crystallography, spectroscopy, and quantum chemical calculations, indicating its potential for various structural and spectroscopic applications in scientific research (Venkatesan et al., 2016).
Molecular Interactions and Network Structures
- Studies have shown that the molecules of this compound are linked into a chain of rings and create a three-dimensional network structure through hydrogen bonds and weak intermolecular interactions, which might be of interest in the study of molecular interactions (Yang et al., 2006).
Radical-Scavenging Activity
- The compound has been analyzed for its radical-scavenging activity, particularly in relation to its nitrogen dioxide radical-scavenging activity. This highlights its potential role in the development or analysis of antioxidants (Kong et al., 2004).
Antimicrobial and Antioxidant Properties
- Studies have indicated the compound's involvement in antimicrobial activities and its characterization in various fatty alkenoates (Rauf & Parveen, 2005).
- It has also been isolated from Lindelofia stylosa and identified as having potent antioxidant properties, which could be significant for pharmacological or biochemistry research (Choudhary et al., 2008).
Materials Science and Photonic Applications
- The compound's derivatives have been studied for their potential use in materials science, such as in the photoalignment of nematic liquid crystals, indicating its utility in photonic applications and display technologies (Hegde et al., 2013).
properties
IUPAC Name |
3-(4-hydroxy-3-methoxyphenyl)prop-2-enoic acid | |
---|---|---|
Details | Computed by LexiChem 2.6.6 (PubChem release 2019.06.18) | |
Source | PubChem | |
URL | https://pubchem.ncbi.nlm.nih.gov | |
Description | Data deposited in or computed by PubChem | |
InChI |
InChI=1S/C10H10O4/c1-14-9-6-7(2-4-8(9)11)3-5-10(12)13/h2-6,11H,1H3,(H,12,13) | |
Details | Computed by InChI 1.0.5 (PubChem release 2019.06.18) | |
Source | PubChem | |
URL | https://pubchem.ncbi.nlm.nih.gov | |
Description | Data deposited in or computed by PubChem | |
InChI Key |
KSEBMYQBYZTDHS-UHFFFAOYSA-N | |
Details | Computed by InChI 1.0.5 (PubChem release 2019.06.18) | |
Source | PubChem | |
URL | https://pubchem.ncbi.nlm.nih.gov | |
Description | Data deposited in or computed by PubChem | |
Canonical SMILES |
COC1=C(C=CC(=C1)C=CC(=O)O)O | |
Details | Computed by OEChem 2.1.5 (PubChem release 2019.06.18) | |
Source | PubChem | |
URL | https://pubchem.ncbi.nlm.nih.gov | |
Description | Data deposited in or computed by PubChem | |
Molecular Formula |
C10H10O4 | |
Details | Computed by PubChem 2.1 (PubChem release 2019.06.18) | |
Source | PubChem | |
URL | https://pubchem.ncbi.nlm.nih.gov | |
Description | Data deposited in or computed by PubChem | |
Related CAS |
97274-61-8 | |
Details | Compound: 2-Propenoic acid, 3-(4-hydroxy-3-methoxyphenyl)-, homopolymer | |
Record name | 2-Propenoic acid, 3-(4-hydroxy-3-methoxyphenyl)-, homopolymer | |
Source | CAS Common Chemistry | |
URL | https://commonchemistry.cas.org/detail?cas_rn=97274-61-8 | |
Description | CAS Common Chemistry is an open community resource for accessing chemical information. Nearly 500,000 chemical substances from CAS REGISTRY cover areas of community interest, including common and frequently regulated chemicals, and those relevant to high school and undergraduate chemistry classes. This chemical information, curated by our expert scientists, is provided in alignment with our mission as a division of the American Chemical Society. | |
Explanation | The data from CAS Common Chemistry is provided under a CC-BY-NC 4.0 license, unless otherwise stated. | |
DSSTOX Substance ID |
DTXSID5040673 | |
Record name | Ferulic acid | |
Source | EPA DSSTox | |
URL | https://comptox.epa.gov/dashboard/DTXSID5040673 | |
Description | DSSTox provides a high quality public chemistry resource for supporting improved predictive toxicology. | |
Molecular Weight |
194.18 g/mol | |
Details | Computed by PubChem 2.1 (PubChem release 2021.05.07) | |
Source | PubChem | |
URL | https://pubchem.ncbi.nlm.nih.gov | |
Description | Data deposited in or computed by PubChem | |
CAS RN |
1135-24-6 | |
Record name | Ferulic acid | |
Source | CAS Common Chemistry | |
URL | https://commonchemistry.cas.org/detail?cas_rn=1135-24-6 | |
Description | CAS Common Chemistry is an open community resource for accessing chemical information. Nearly 500,000 chemical substances from CAS REGISTRY cover areas of community interest, including common and frequently regulated chemicals, and those relevant to high school and undergraduate chemistry classes. This chemical information, curated by our expert scientists, is provided in alignment with our mission as a division of the American Chemical Society. | |
Explanation | The data from CAS Common Chemistry is provided under a CC-BY-NC 4.0 license, unless otherwise stated. | |
Record name | Ferulic acid | |
Source | EPA DSSTox | |
URL | https://comptox.epa.gov/dashboard/DTXSID5040673 | |
Description | DSSTox provides a high quality public chemistry resource for supporting improved predictive toxicology. | |
Synthesis routes and methods I
Procedure details
Synthesis routes and methods II
Procedure details
Retrosynthesis Analysis
AI-Powered Synthesis Planning: Our tool employs the Template_relevance Pistachio, Template_relevance Bkms_metabolic, Template_relevance Pistachio_ringbreaker, Template_relevance Reaxys, Template_relevance Reaxys_biocatalysis model, leveraging a vast database of chemical reactions to predict feasible synthetic routes.
One-Step Synthesis Focus: Specifically designed for one-step synthesis, it provides concise and direct routes for your target compounds, streamlining the synthesis process.
Accurate Predictions: Utilizing the extensive PISTACHIO, BKMS_METABOLIC, PISTACHIO_RINGBREAKER, REAXYS, REAXYS_BIOCATALYSIS database, our tool offers high-accuracy predictions, reflecting the latest in chemical research and data.
Strategy Settings
Precursor scoring | Relevance Heuristic |
---|---|
Min. plausibility | 0.01 |
Model | Template_relevance |
Template Set | Pistachio/Bkms_metabolic/Pistachio_ringbreaker/Reaxys/Reaxys_biocatalysis |
Top-N result to add to graph | 6 |
Feasible Synthetic Routes
Disclaimer and Information on In-Vitro Research Products
Please be aware that all articles and product information presented on BenchChem are intended solely for informational purposes. The products available for purchase on BenchChem are specifically designed for in-vitro studies, which are conducted outside of living organisms. In-vitro studies, derived from the Latin term "in glass," involve experiments performed in controlled laboratory settings using cells or tissues. It is important to note that these products are not categorized as medicines or drugs, and they have not received approval from the FDA for the prevention, treatment, or cure of any medical condition, ailment, or disease. We must emphasize that any form of bodily introduction of these products into humans or animals is strictly prohibited by law. It is essential to adhere to these guidelines to ensure compliance with legal and ethical standards in research and experimentation.