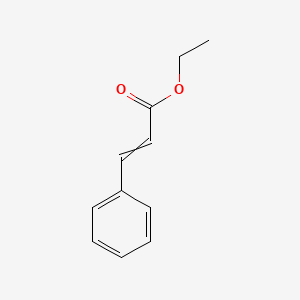
Ethyl 3-phenylprop-2-enoate
Overview
Description
{11})H({12})O(_{2}). It is a colorless to pale yellow liquid with a sweet, balsamic odor reminiscent of cinnamon and strawberries. This compound is widely used in the flavor and fragrance industry due to its pleasant aroma and is also found in various natural sources such as styrax and galangal oil .
Preparation Methods
Synthetic Routes and Reaction Conditions: Ethyl 3-phenylprop-2-enoate is typically synthesized through the esterification of cinnamic acid with ethanol in the presence of an acid catalyst such as sulfuric acid. The reaction is carried out under reflux conditions to ensure complete conversion of the reactants to the ester product.
Industrial Production Methods: In industrial settings, the production of this compound often involves the use of continuous flow reactors to optimize yield and efficiency. The process includes the distillation of the product to achieve high purity levels. The reaction conditions are carefully controlled to prevent polymerization and degradation of the product .
Chemical Reactions Analysis
Types of Reactions: Ethyl 3-phenylprop-2-enoate undergoes various chemical reactions, including:
Oxidation: It can be oxidized to cinnamic acid using oxidizing agents like potassium permanganate.
Reduction: Reduction of this compound can yield ethyl 3-phenylpropanoate using hydrogen gas in the presence of a palladium catalyst.
Substitution: It can participate in nucleophilic substitution reactions, where the ethoxy group can be replaced by other nucleophiles.
Common Reagents and Conditions:
Oxidation: Potassium permanganate in an aqueous medium.
Reduction: Hydrogen gas with a palladium catalyst.
Substitution: Nucleophiles such as amines or thiols under basic conditions.
Major Products Formed:
Oxidation: Cinnamic acid.
Reduction: Ethyl 3-phenylpropanoate.
Substitution: Various substituted cinnamates depending on the nucleophile used.
Scientific Research Applications
Ethyl 3-phenylprop-2-enoate has diverse applications in scientific research:
Chemistry: It is used as a starting material for the synthesis of various organic compounds and as a reagent in organic synthesis.
Biology: It is employed in studies involving cell signaling and metabolic pathways due to its ability to modulate enzyme activity.
Medicine: Research has explored its potential anti-inflammatory and antimicrobial properties, making it a candidate for pharmaceutical development.
Mechanism of Action
The mechanism by which ethyl 3-phenylprop-2-enoate exerts its effects involves its interaction with various molecular targets. For instance, its antimicrobial activity is attributed to its ability to disrupt microbial cell membranes, leading to cell lysis. In anti-inflammatory applications, it inhibits the activity of enzymes such as cyclooxygenase, reducing the production of pro-inflammatory mediators.
Comparison with Similar Compounds
Ethyl 3-phenylprop-2-enoate can be compared with other cinnamate esters such as methyl cinnamate and benzyl cinnamate:
Methyl Cinnamate: Similar in structure but with a methyl group instead of an ethyl group. It has a slightly different aroma profile and solubility characteristics.
Benzyl Cinnamate: Contains a benzyl group, making it more hydrophobic and altering its fragrance and chemical reactivity.
Uniqueness: this compound is unique due to its balanced solubility in organic solvents and its specific aroma profile, which makes it particularly valuable in the flavor and fragrance industry.
Properties
IUPAC Name |
ethyl 3-phenylprop-2-enoate | |
---|---|---|
Details | Computed by Lexichem TK 2.7.0 (PubChem release 2021.05.07) | |
Source | PubChem | |
URL | https://pubchem.ncbi.nlm.nih.gov | |
Description | Data deposited in or computed by PubChem | |
InChI |
InChI=1S/C11H12O2/c1-2-13-11(12)9-8-10-6-4-3-5-7-10/h3-9H,2H2,1H3 | |
Details | Computed by InChI 1.0.6 (PubChem release 2021.05.07) | |
Source | PubChem | |
URL | https://pubchem.ncbi.nlm.nih.gov | |
Description | Data deposited in or computed by PubChem | |
InChI Key |
KBEBGUQPQBELIU-UHFFFAOYSA-N | |
Details | Computed by InChI 1.0.6 (PubChem release 2021.05.07) | |
Source | PubChem | |
URL | https://pubchem.ncbi.nlm.nih.gov | |
Description | Data deposited in or computed by PubChem | |
Canonical SMILES |
CCOC(=O)C=CC1=CC=CC=C1 | |
Details | Computed by OEChem 2.3.0 (PubChem release 2021.05.07) | |
Source | PubChem | |
URL | https://pubchem.ncbi.nlm.nih.gov | |
Description | Data deposited in or computed by PubChem | |
Molecular Formula |
C11H12O2 | |
Details | Computed by PubChem 2.1 (PubChem release 2021.05.07) | |
Source | PubChem | |
URL | https://pubchem.ncbi.nlm.nih.gov | |
Description | Data deposited in or computed by PubChem | |
DSSTOX Substance ID |
DTXSID9022520 | |
Record name | Ethyl cinnamate | |
Source | EPA DSSTox | |
URL | https://comptox.epa.gov/dashboard/DTXSID9022520 | |
Description | DSSTox provides a high quality public chemistry resource for supporting improved predictive toxicology. | |
Molecular Weight |
176.21 g/mol | |
Details | Computed by PubChem 2.1 (PubChem release 2021.05.07) | |
Source | PubChem | |
URL | https://pubchem.ncbi.nlm.nih.gov | |
Description | Data deposited in or computed by PubChem | |
CAS No. |
103-36-6 | |
Record name | Ethyl cinnamate | |
Source | CAS Common Chemistry | |
URL | https://commonchemistry.cas.org/detail?cas_rn=103-36-6 | |
Description | CAS Common Chemistry is an open community resource for accessing chemical information. Nearly 500,000 chemical substances from CAS REGISTRY cover areas of community interest, including common and frequently regulated chemicals, and those relevant to high school and undergraduate chemistry classes. This chemical information, curated by our expert scientists, is provided in alignment with our mission as a division of the American Chemical Society. | |
Explanation | The data from CAS Common Chemistry is provided under a CC-BY-NC 4.0 license, unless otherwise stated. | |
Record name | Ethyl cinnamate | |
Source | EPA DSSTox | |
URL | https://comptox.epa.gov/dashboard/DTXSID9022520 | |
Description | DSSTox provides a high quality public chemistry resource for supporting improved predictive toxicology. | |
Synthesis routes and methods I
Procedure details
Synthesis routes and methods II
Procedure details
Synthesis routes and methods III
Procedure details
Synthesis routes and methods IV
Procedure details
Retrosynthesis Analysis
AI-Powered Synthesis Planning: Our tool employs the Template_relevance Pistachio, Template_relevance Bkms_metabolic, Template_relevance Pistachio_ringbreaker, Template_relevance Reaxys, Template_relevance Reaxys_biocatalysis model, leveraging a vast database of chemical reactions to predict feasible synthetic routes.
One-Step Synthesis Focus: Specifically designed for one-step synthesis, it provides concise and direct routes for your target compounds, streamlining the synthesis process.
Accurate Predictions: Utilizing the extensive PISTACHIO, BKMS_METABOLIC, PISTACHIO_RINGBREAKER, REAXYS, REAXYS_BIOCATALYSIS database, our tool offers high-accuracy predictions, reflecting the latest in chemical research and data.
Strategy Settings
Precursor scoring | Relevance Heuristic |
---|---|
Min. plausibility | 0.01 |
Model | Template_relevance |
Template Set | Pistachio/Bkms_metabolic/Pistachio_ringbreaker/Reaxys/Reaxys_biocatalysis |
Top-N result to add to graph | 6 |
Feasible Synthetic Routes
Disclaimer and Information on In-Vitro Research Products
Please be aware that all articles and product information presented on BenchChem are intended solely for informational purposes. The products available for purchase on BenchChem are specifically designed for in-vitro studies, which are conducted outside of living organisms. In-vitro studies, derived from the Latin term "in glass," involve experiments performed in controlled laboratory settings using cells or tissues. It is important to note that these products are not categorized as medicines or drugs, and they have not received approval from the FDA for the prevention, treatment, or cure of any medical condition, ailment, or disease. We must emphasize that any form of bodily introduction of these products into humans or animals is strictly prohibited by law. It is essential to adhere to these guidelines to ensure compliance with legal and ethical standards in research and experimentation.