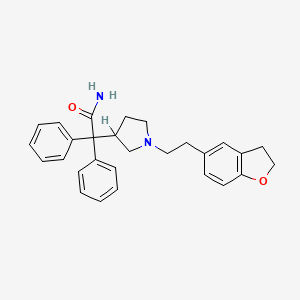
2-(1-(2-(2,3-Dihydrobenzofuran-5-yl)ethyl)pyrrolidin-3-yl)-2,2-diphenylacetamide
Overview
Description
Darifenacin-d4 is a deuterated form of Darifenacin, a medication primarily used to treat urinary incontinence by blocking M3 muscarinic acetylcholine receptors. The deuterated form, Darifenacin-d4, is often used as an internal standard in various analytical methods due to its stable isotope labeling .
Preparation Methods
Synthetic Routes and Reaction Conditions
The synthesis of Darifenacin-d4 involves the incorporation of deuterium atoms into the Darifenacin molecule. This process typically includes the use of deuterated reagents and solvents. One common method involves the reaction of Darifenacin with deuterated benzofuran and pyrrolidine derivatives under controlled conditions .
Industrial Production Methods
Industrial production of Darifenacin-d4 follows similar synthetic routes but on a larger scale. The process involves rigorous quality control measures to ensure the purity and consistency of the final product. The use of high-purity deuterated reagents and advanced purification techniques, such as chromatography, is essential .
Chemical Reactions Analysis
Types of Reactions
Darifenacin-d4 undergoes various chemical reactions, including:
Oxidation: Involves the addition of oxygen or the removal of hydrogen.
Reduction: Involves the addition of hydrogen or the removal of oxygen.
Substitution: Involves the replacement of one atom or group of atoms with another.
Common Reagents and Conditions
Oxidation: Common oxidizing agents include potassium permanganate and hydrogen peroxide.
Reduction: Common reducing agents include lithium aluminum hydride and sodium borohydride.
Substitution: Common reagents include halogens and nucleophiles under various conditions.
Major Products Formed
The major products formed from these reactions depend on the specific conditions and reagents used. For example, oxidation may yield hydroxylated derivatives, while reduction may produce deuterated alcohols .
Scientific Research Applications
Darifenacin-d4 has several scientific research applications, including:
Chemistry: Used as an internal standard in mass spectrometry and nuclear magnetic resonance (NMR) spectroscopy.
Biology: Employed in studies involving muscarinic acetylcholine receptors to understand their role in various physiological processes.
Medicine: Used in pharmacokinetic studies to track the metabolism and distribution of Darifenacin in the body.
Industry: Utilized in the development and validation of analytical methods for quality control in pharmaceutical production
Mechanism of Action
Darifenacin-d4, like its non-deuterated counterpart, works by selectively blocking M3 muscarinic acetylcholine receptors. These receptors are primarily responsible for bladder muscle contractions. By inhibiting these receptors, Darifenacin-d4 reduces the urgency to urinate. The molecular targets include the M3 receptors, and the pathways involved are related to the inhibition of acetylcholine-mediated signaling .
Comparison with Similar Compounds
Similar Compounds
Oxybutynin: Another muscarinic receptor antagonist used to treat overactive bladder.
Tolterodine: A competitive muscarinic receptor antagonist with similar applications.
Solifenacin: Selectively targets M3 receptors, similar to Darifenacin.
Uniqueness
Darifenacin-d4 is unique due to its deuterium labeling, which provides enhanced stability and allows for precise analytical measurements. This makes it particularly valuable in research and industrial applications where accurate quantification is essential .
Properties
IUPAC Name |
2-[1-[2-(2,3-dihydro-1-benzofuran-5-yl)ethyl]pyrrolidin-3-yl]-2,2-diphenylacetamide | |
---|---|---|
Details | Computed by Lexichem TK 2.7.0 (PubChem release 2021.05.07) | |
Source | PubChem | |
URL | https://pubchem.ncbi.nlm.nih.gov | |
Description | Data deposited in or computed by PubChem | |
InChI |
InChI=1S/C28H30N2O2/c29-27(31)28(23-7-3-1-4-8-23,24-9-5-2-6-10-24)25-14-17-30(20-25)16-13-21-11-12-26-22(19-21)15-18-32-26/h1-12,19,25H,13-18,20H2,(H2,29,31) | |
Details | Computed by InChI 1.0.6 (PubChem release 2021.05.07) | |
Source | PubChem | |
URL | https://pubchem.ncbi.nlm.nih.gov | |
Description | Data deposited in or computed by PubChem | |
InChI Key |
HXGBXQDTNZMWGS-UHFFFAOYSA-N | |
Details | Computed by InChI 1.0.6 (PubChem release 2021.05.07) | |
Source | PubChem | |
URL | https://pubchem.ncbi.nlm.nih.gov | |
Description | Data deposited in or computed by PubChem | |
Canonical SMILES |
C1CN(CC1C(C2=CC=CC=C2)(C3=CC=CC=C3)C(=O)N)CCC4=CC5=C(C=C4)OCC5 | |
Details | Computed by OEChem 2.3.0 (PubChem release 2021.05.07) | |
Source | PubChem | |
URL | https://pubchem.ncbi.nlm.nih.gov | |
Description | Data deposited in or computed by PubChem | |
Molecular Formula |
C28H30N2O2 | |
Details | Computed by PubChem 2.1 (PubChem release 2021.05.07) | |
Source | PubChem | |
URL | https://pubchem.ncbi.nlm.nih.gov | |
Description | Data deposited in or computed by PubChem | |
DSSTOX Substance ID |
DTXSID50861320 | |
Record name | (±)-Darifenacin | |
Source | EPA DSSTox | |
URL | https://comptox.epa.gov/dashboard/DTXSID50861320 | |
Description | DSSTox provides a high quality public chemistry resource for supporting improved predictive toxicology. | |
Molecular Weight |
426.5 g/mol | |
Details | Computed by PubChem 2.1 (PubChem release 2021.05.07) | |
Source | PubChem | |
URL | https://pubchem.ncbi.nlm.nih.gov | |
Description | Data deposited in or computed by PubChem | |
CAS No. |
133033-93-9, 133099-04-4 | |
Record name | (±)-Darifenacin | |
Source | EPA DSSTox | |
URL | https://comptox.epa.gov/dashboard/DTXSID50861320 | |
Description | DSSTox provides a high quality public chemistry resource for supporting improved predictive toxicology. | |
Record name | 3-Pyrrolidineacetamide, 1-[2-(2,3-dihydro-5-benzofuranyl)ethyl]-α,α-diphenyl-, (3S) | |
Source | European Chemicals Agency (ECHA) | |
URL | https://echa.europa.eu/information-on-chemicals | |
Description | The European Chemicals Agency (ECHA) is an agency of the European Union which is the driving force among regulatory authorities in implementing the EU's groundbreaking chemicals legislation for the benefit of human health and the environment as well as for innovation and competitiveness. | |
Explanation | Use of the information, documents and data from the ECHA website is subject to the terms and conditions of this Legal Notice, and subject to other binding limitations provided for under applicable law, the information, documents and data made available on the ECHA website may be reproduced, distributed and/or used, totally or in part, for non-commercial purposes provided that ECHA is acknowledged as the source: "Source: European Chemicals Agency, http://echa.europa.eu/". Such acknowledgement must be included in each copy of the material. ECHA permits and encourages organisations and individuals to create links to the ECHA website under the following cumulative conditions: Links can only be made to webpages that provide a link to the Legal Notice page. | |
Synthesis routes and methods
Procedure details
Retrosynthesis Analysis
AI-Powered Synthesis Planning: Our tool employs the Template_relevance Pistachio, Template_relevance Bkms_metabolic, Template_relevance Pistachio_ringbreaker, Template_relevance Reaxys, Template_relevance Reaxys_biocatalysis model, leveraging a vast database of chemical reactions to predict feasible synthetic routes.
One-Step Synthesis Focus: Specifically designed for one-step synthesis, it provides concise and direct routes for your target compounds, streamlining the synthesis process.
Accurate Predictions: Utilizing the extensive PISTACHIO, BKMS_METABOLIC, PISTACHIO_RINGBREAKER, REAXYS, REAXYS_BIOCATALYSIS database, our tool offers high-accuracy predictions, reflecting the latest in chemical research and data.
Strategy Settings
Precursor scoring | Relevance Heuristic |
---|---|
Min. plausibility | 0.01 |
Model | Template_relevance |
Template Set | Pistachio/Bkms_metabolic/Pistachio_ringbreaker/Reaxys/Reaxys_biocatalysis |
Top-N result to add to graph | 6 |
Feasible Synthetic Routes
Disclaimer and Information on In-Vitro Research Products
Please be aware that all articles and product information presented on BenchChem are intended solely for informational purposes. The products available for purchase on BenchChem are specifically designed for in-vitro studies, which are conducted outside of living organisms. In-vitro studies, derived from the Latin term "in glass," involve experiments performed in controlled laboratory settings using cells or tissues. It is important to note that these products are not categorized as medicines or drugs, and they have not received approval from the FDA for the prevention, treatment, or cure of any medical condition, ailment, or disease. We must emphasize that any form of bodily introduction of these products into humans or animals is strictly prohibited by law. It is essential to adhere to these guidelines to ensure compliance with legal and ethical standards in research and experimentation.