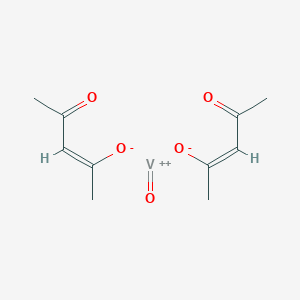
Vanadyl acetylacetonate
Overview
Description
Vanadyl acetylacetonate ([VO(acac)₂]) is a coordination complex of vanadium(IV) with acetylacetone (acac) ligands. The compound’s octahedral geometry and redox-active vanadyl (VO²⁺) core contribute to its reactivity in biological and chemical systems. Its lipophilic acetylacetonate ligands enhance membrane permeability, making it more effective in cellular uptake compared to inorganic vanadium salts like vanadyl sulfate (VOSO₄) .
Preparation Methods
Vanadyl acetylacetonate can be synthesized through several methods. One common synthetic route involves the reaction of vanadyl sulfate (VOSO₄) with acetylacetone (Hacac) under acidic conditions, producing this compound and sulfuric acid as a byproduct : [ \text{VOSO}_4 + 2 \text{Hacac} \rightarrow \text{VO(acac)}_2 + \text{H}_2\text{SO}_4 ]
Another method involves a redox reaction starting with vanadium pentoxide (V₂O₅), where some acetylacetone is oxidized to 2,3,4-pentanetrione . Industrial production methods typically follow similar synthetic routes but are optimized for larger-scale production and higher yields .
Chemical Reactions Analysis
Vanadyl acetylacetonate undergoes various chemical reactions, including oxidation, reduction, and substitution reactions. It is commonly used as a catalyst in organic chemistry, particularly for the epoxidation of allylic alcohols by tert-butyl hydroperoxide (TBHP) . The VO(acac)₂-TBHP system selectively epoxidizes allylic alcohols, leaving other alkenes untouched .
In addition to epoxidation, this compound can participate in Mannich reactions, sulfoxidation of alkanes, and asymmetric oxidation of disulfides . Common reagents and conditions used in these reactions include TBHP, pyridine, and methylamine . The major products formed from these reactions depend on the specific substrates and reaction conditions employed.
Scientific Research Applications
Medicinal Applications
1.1 Anticancer Activity
Vanadyl acetylacetonate has been investigated for its potential as an anticancer agent. Studies have shown that vanadyl complexes exhibit antiproliferative effects on various cancer cell lines, including colorectal adenocarcinoma. The mechanism involves the modulation of cell cycle progression and activation of mitogen-activated protein kinases (MAPKs). For instance, one study demonstrated that treatment with vanadyl complexes induced a block in the S-G2/M phase of the cell cycle in specific cell lines, leading to decreased proliferation rates .
1.2 Diabetes Management
Research indicates that vanadyl compounds, including VO(acac)₂, possess insulin-mimetic properties that can aid in diabetes management. They enhance glucose uptake and improve insulin sensitivity, making them potential candidates for developing new antidiabetic therapies. In vivo studies have shown that these complexes can lower blood glucose levels in diabetic models .
1.3 Alzheimer's Disease Research
This compound has also been studied for its neuroprotective effects in Alzheimer's disease models. In APP/PS1 transgenic mice, VO(acac)₂ administration was linked to reduced amyloid-beta (Aβ) plaque formation and improved cognitive functions. The timing of administration was crucial; earlier intervention led to better outcomes in Aβ clearance compared to later treatment .
Imaging Applications
2.1 Cancer Imaging
Recent advancements have utilized this compound as a radiotracer for cancer imaging. Specifically, V-48 labeled VO(acac)₂ has been employed in dual-modality PET/MRI scanning to enhance image contrast for early-stage cancer detection. Studies have demonstrated its efficacy in improving the biodistribution and uptake of radiotracers in animal models, which could revolutionize early cancer diagnosis strategies .
Material Science Applications
3.1 Electrochemical Studies
This compound has been explored in electrochemical applications, particularly its reduction processes in acetonitrile solutions. Research utilizing cyclic voltammetry and polarography has provided insights into its redox behavior, which is essential for developing new materials for batteries and sensors .
Table 1: Summary of Biological Activities of this compound
Mechanism of Action
The mechanism of action of vanadyl acetylacetonate involves its ability to mimic insulin and modulate various biochemical pathways. It can stimulate the phosphorylation of PKB/Akt and GSK-3, enhancing the insulin signaling pathway and promoting glucose metabolism . This compound also upregulates peroxisome-proliferator-activated receptor γ (PPARγ) and adiponectin expression in differentiated adipocytes, contributing to its antidiabetic effects .
Furthermore, this compound can inhibit protein tyrosine phosphatase 1B (PTP1B), which enhances the phosphorylation of insulin receptor substrate 1 and activation of phosphatidylinositol 3-kinase (PI3K)-Akt signal transduction . This mechanism may explain its potential therapeutic benefits for diabetes and cancer.
Comparison with Similar Compounds
Comparison with Similar Vanadium Compounds
Antidiabetic Activity
Vanadyl acetylacetonate exhibits superior glycemic control compared to other vanadium complexes. Key findings include:
Table 1: Comparative Antidiabetic Efficacy in Diabetic Rats
- Mechanistic Differences: this compound acts as a mixed non-competitive/uncompetitive inhibitor of glucose-6-phosphatase, while vanadyl sulfate is a competitive inhibitor . In diabetic rat hepatocytes, this compound restores glycolytic pathways by upregulating glucokinase and downregulating phosphoenolpyruvate carboxykinase (PEPCK), outperforming vanadyl sulfate .
Antiproliferative Activity
This compound derivatives show ligand-dependent antitumor effects:
- Complexes with asymmetric β-dicarbonyl ligands exhibit enhanced cytotoxicity against tumor cell lines compared to symmetric ligands. For example, fluorinated derivatives (Complexes c and d) demonstrated higher antiproliferative activity due to improved cellular localization and interaction with MAPK signaling pathways .
- Comparative Advantage : The acetylacetonate ligand’s electron-withdrawing properties stabilize the vanadyl moiety, enhancing redox activity and DNA interaction .
Stability and Redox Behavior
- Redox Flow Batteries: this compound can be reduced to vanadium acetylacetonate ([V(acac)₃]), but this process is irreversible under moist conditions due to re-oxidation to VO(acac)₂. This contrasts with more stable derivatives like vanadium acetylacetonate, which require extreme potentials for reduction .
- Thermal Decomposition : this compound decomposes at 200–300°C, forming vanadium oxides and carbonaceous residues. Its decomposition pathway is more predictable than vanadyl sulfate, which releases SO₃ at lower temperatures .
Key Research Findings and Data
Table 2: Comparative Biochemical and Physical Properties
Property | This compound | Vanadyl Sulfate | Bis(maltolato)oxovanadium |
---|---|---|---|
Solubility in Water | Low | High | Moderate |
IC₅₀ for Glucose-6-Phosphatase | 200 µM | 48 µM | Not reported |
Thermal Stability (°C) | 200–300 | <150 | 180–250 |
Bioavailability (Oral) | High | Low | Moderate |
Q & A
Basic Research Questions
Q. What is the coordination geometry of vanadium in VO(acac)₂, and how is it experimentally determined?
The vanadium center in VO(acac)₂ adopts a square pyramidal geometry with five oxygen ligands. The apical position is occupied by the vanadyl oxygen (V=O bond length: 1.56 Å), while the four equatorial positions are coordinated by acetylacetonate oxygen atoms (V–O distances: 1.96–1.98 Å). This structure was confirmed via single-crystal X-ray diffraction , which revealed triclinic symmetry (space group P1) and discrete molecular packing . Computational modeling of bond angles and distances further supports the square pyramidal arrangement over trigonal bipyramidal alternatives .
Q. What spectroscopic methods are commonly used to characterize VO(acac)₂?
Key techniques include:
- Infrared (IR) Spectroscopy : Identifies the V=O stretching vibration at ~996 cm⁻¹ and acetylacetonate ligand vibrations (C–O, C–C) between 300–780 cm⁻¹ .
- Electron Paramagnetic Resonance (EPR) : Measures hyperfine coupling constants (e.g., 51V interaction at ~160 G) to probe electronic structure and solvent interactions .
- UV-Vis Spectroscopy : Reveals ligand-to-metal charge transfer (LMCT) bands in the 10,000–50,000 cm⁻¹ range, with shifts dependent on solvent polarity .
Q. How is VO(acac)₂ synthesized, and what purity considerations are critical?
VO(acac)₂ is typically synthesized by reacting vanadyl sulfate (VOSO₄) with acetylacetone in aqueous or alcoholic media. Purification involves recrystallization from non-polar solvents (e.g., benzene) to achieve >95% purity. Thermal stability must be monitored via thermogravimetric analysis (TGA) , as decomposition begins at ~235°C .
Q. What safety protocols are essential when handling VO(acac)₂?
VO(acac)₂ is toxic upon ingestion, inhalation, or skin contact. Safety measures include:
- Personal Protective Equipment (PPE) : Lab coat, gloves, and eye protection.
- Spill Management : Use inert absorbents (e.g., sand) and avoid water to prevent dispersion.
- First Aid : Immediate rinsing with water for eye/skin exposure and medical consultation .
Advanced Research Questions
Q. How does solvent composition influence the EPR parameters of VO(acac)₂?
Solvent polarity and viscosity modulate the rotational correlation time (τR) and hyperfine coupling. For example, in ethanol-CCl₄ mixtures, τR follows a modified Debye equation:
where is solvent viscosity and is a solvent-specific coupling parameter. Ethanol increases linewidth broadening due to stronger hydrogen bonding, while non-polar solvents like CCl₄ reduce rotational friction .
Q. What reaction pathways govern VO(acac)₂’s role in stereospecific epoxidation?
VO(acac)₂ catalyzes allylic alcohol epoxidation via a radical mechanism . Key steps include:
- Substrate Activation : Hydroperoxides (e.g., tert-butyl hydroperoxide) oxidize V(IV) to V(V).
- Oxygen Transfer : The V(V)-peroxo intermediate transfers an oxygen atom to the alkene, forming an epoxide with >90% stereoselectivity in aromatic solvents (e.g., toluene) .
- Optimization : Catalyst loading (1–10 wt%), solvent polarity, and temperature (20–60°C) are critical for yield and enantiomeric excess .
Q. How does thermal decomposition of VO(acac)₂ enable nanocrystal synthesis?
Heating VO(acac)₂ under inert atmospheres (e.g., N₂) at 250–300°C induces ligand dissociation, forming vanadium oxide (VOx) nanocrystals . Reaction monitoring via attenuated total reflectance FTIR (ATR-FTIR) shows sequential loss of acetylacetonate C–O and C–C bonds. The nanocrystals exhibit quantum confinement effects, with optical bandgaps tunable via decomposition duration and temperature .
Q. What contradictions exist in interpreting VO(acac)₂’s electronic spectra?
Discrepancies arise in correlating UV-Vis bands with d-orbital transitions. For example:
- Ballhausen-Gray Model : Assigns LMCT bands to transitions, but fails to explain solvent-induced shifts.
- Revised Assignments : Later studies attribute ~37,500 cm⁻¹ bands to ligand-centered transitions, supported by vapor-phase spectra of fluorinated analogs (e.g., VO(hfac)₂) .
Q. How does VO(acac)₂ interact with biological systems, and what methodological challenges arise?
VO(acac)₂ mimics insulin by inhibiting glycogen synthase kinase-3 (GSK-3) and activating insulin receptor substrates. In vivo studies (e.g., rat femoral fracture models) require:
Properties
IUPAC Name |
(Z)-4-oxopent-2-en-2-olate;oxovanadium(2+) | |
---|---|---|
Details | Computed by LexiChem 2.6.6 (PubChem release 2019.06.18) | |
Source | PubChem | |
URL | https://pubchem.ncbi.nlm.nih.gov | |
Description | Data deposited in or computed by PubChem | |
InChI |
InChI=1S/2C5H8O2.O.V/c2*1-4(6)3-5(2)7;;/h2*3,6H,1-2H3;;/q;;;+2/p-2/b2*4-3-;; | |
Details | Computed by InChI 1.0.5 (PubChem release 2019.06.18) | |
Source | PubChem | |
URL | https://pubchem.ncbi.nlm.nih.gov | |
Description | Data deposited in or computed by PubChem | |
InChI Key |
JFHJZWAQYMGNBE-SUKNRPLKSA-L | |
Details | Computed by InChI 1.0.5 (PubChem release 2019.06.18) | |
Source | PubChem | |
URL | https://pubchem.ncbi.nlm.nih.gov | |
Description | Data deposited in or computed by PubChem | |
Canonical SMILES |
CC(=CC(=O)C)[O-].CC(=CC(=O)C)[O-].O=[V+2] | |
Details | Computed by OEChem 2.1.5 (PubChem release 2019.06.18) | |
Source | PubChem | |
URL | https://pubchem.ncbi.nlm.nih.gov | |
Description | Data deposited in or computed by PubChem | |
Isomeric SMILES |
C/C(=C/C(=O)C)/[O-].C/C(=C/C(=O)C)/[O-].O=[V+2] | |
Details | Computed by OEChem 2.1.5 (PubChem release 2019.06.18) | |
Source | PubChem | |
URL | https://pubchem.ncbi.nlm.nih.gov | |
Description | Data deposited in or computed by PubChem | |
Molecular Formula |
C10H14O5V | |
Details | Computed by PubChem 2.1 (PubChem release 2019.06.18) | |
Source | PubChem | |
URL | https://pubchem.ncbi.nlm.nih.gov | |
Description | Data deposited in or computed by PubChem | |
Molecular Weight |
265.16 g/mol | |
Details | Computed by PubChem 2.1 (PubChem release 2021.05.07) | |
Source | PubChem | |
URL | https://pubchem.ncbi.nlm.nih.gov | |
Description | Data deposited in or computed by PubChem | |
Physical Description |
Blue or green powder; Slightly soluble in water; [MSDSonline] | |
Record name | Vanadyl bis(acetylacetonate) | |
Source | Haz-Map, Information on Hazardous Chemicals and Occupational Diseases | |
URL | https://haz-map.com/Agents/8234 | |
Description | Haz-Map® is an occupational health database designed for health and safety professionals and for consumers seeking information about the adverse effects of workplace exposures to chemical and biological agents. | |
Explanation | Copyright (c) 2022 Haz-Map(R). All rights reserved. Unless otherwise indicated, all materials from Haz-Map are copyrighted by Haz-Map(R). No part of these materials, either text or image may be used for any purpose other than for personal use. Therefore, reproduction, modification, storage in a retrieval system or retransmission, in any form or by any means, electronic, mechanical or otherwise, for reasons other than personal use, is strictly prohibited without prior written permission. | |
CAS No. |
3153-26-2 | |
Record name | Vanadium, oxobis(2,4-pentanedionato-.kappa.O2,.kappa.O4)-, (SP-5-21)- | |
Source | EPA Chemicals under the TSCA | |
URL | https://www.epa.gov/chemicals-under-tsca | |
Description | EPA Chemicals under the Toxic Substances Control Act (TSCA) collection contains information on chemicals and their regulations under TSCA, including non-confidential content from the TSCA Chemical Substance Inventory and Chemical Data Reporting. | |
Record name | Oxobis(pentane-2,4-dionato-O,O')vanadium | |
Source | European Chemicals Agency (ECHA) | |
URL | https://echa.europa.eu/substance-information/-/substanceinfo/100.019.628 | |
Description | The European Chemicals Agency (ECHA) is an agency of the European Union which is the driving force among regulatory authorities in implementing the EU's groundbreaking chemicals legislation for the benefit of human health and the environment as well as for innovation and competitiveness. | |
Explanation | Use of the information, documents and data from the ECHA website is subject to the terms and conditions of this Legal Notice, and subject to other binding limitations provided for under applicable law, the information, documents and data made available on the ECHA website may be reproduced, distributed and/or used, totally or in part, for non-commercial purposes provided that ECHA is acknowledged as the source: "Source: European Chemicals Agency, http://echa.europa.eu/". Such acknowledgement must be included in each copy of the material. ECHA permits and encourages organisations and individuals to create links to the ECHA website under the following cumulative conditions: Links can only be made to webpages that provide a link to the Legal Notice page. | |
Retrosynthesis Analysis
AI-Powered Synthesis Planning: Our tool employs the Template_relevance Pistachio, Template_relevance Bkms_metabolic, Template_relevance Pistachio_ringbreaker, Template_relevance Reaxys, Template_relevance Reaxys_biocatalysis model, leveraging a vast database of chemical reactions to predict feasible synthetic routes.
One-Step Synthesis Focus: Specifically designed for one-step synthesis, it provides concise and direct routes for your target compounds, streamlining the synthesis process.
Accurate Predictions: Utilizing the extensive PISTACHIO, BKMS_METABOLIC, PISTACHIO_RINGBREAKER, REAXYS, REAXYS_BIOCATALYSIS database, our tool offers high-accuracy predictions, reflecting the latest in chemical research and data.
Strategy Settings
Precursor scoring | Relevance Heuristic |
---|---|
Min. plausibility | 0.01 |
Model | Template_relevance |
Template Set | Pistachio/Bkms_metabolic/Pistachio_ringbreaker/Reaxys/Reaxys_biocatalysis |
Top-N result to add to graph | 6 |
Feasible Synthetic Routes
Disclaimer and Information on In-Vitro Research Products
Please be aware that all articles and product information presented on BenchChem are intended solely for informational purposes. The products available for purchase on BenchChem are specifically designed for in-vitro studies, which are conducted outside of living organisms. In-vitro studies, derived from the Latin term "in glass," involve experiments performed in controlled laboratory settings using cells or tissues. It is important to note that these products are not categorized as medicines or drugs, and they have not received approval from the FDA for the prevention, treatment, or cure of any medical condition, ailment, or disease. We must emphasize that any form of bodily introduction of these products into humans or animals is strictly prohibited by law. It is essential to adhere to these guidelines to ensure compliance with legal and ethical standards in research and experimentation.