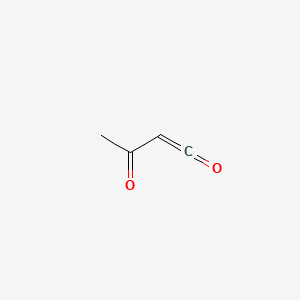
But-1-ene-1,3-dione
Overview
Description
But-1-ene-1,3-dione (CAS 691-45-2) is an α,β-unsaturated diketone with the molecular formula C₄H₄O₂ and a molecular weight of 84.07 g/mol . Its structure features conjugated carbonyl groups at positions 1 and 3, separated by a double bond (Fig. 1). This electronic configuration renders it highly reactive, particularly in cycloaddition and nucleophilic addition reactions. It is used as a precursor in synthesizing heterocyclic compounds, such as 2-acetyloxyacetyl chloride ethyl ester . Limited physical property data (e.g., melting/boiling points) are available in open literature, but its reactivity is well-documented in studies involving ketene intermediates and cyclization reactions .
Fig. 1: Structure of this compound.
Preparation Methods
Synthetic Routes and Reaction Conditions: But-1-ene-1,3-dione can be synthesized through various methods. One common method involves the oxidative dehydrogenation of but-1-ene using catalysts such as vanadium-magnesium complex oxides . Another method includes the cleavage of acetic acid at high temperatures (750-780°C) in the presence of triethyl phosphate to produce ketene, which is then polymerized at low temperatures (8-10°C) to form this compound .
Industrial Production Methods: Industrial production of this compound often involves the use of oxidative dehydrogenation processes due to their efficiency and selectivity. The use of metal oxide catalysts, such as vanadium-magnesium, has been shown to enhance the yield and selectivity of the desired product .
Chemical Reactions Analysis
Types of Reactions: But-1-ene-1,3-dione undergoes various chemical reactions, including:
Oxidation: It can be oxidized to form buta-1,3-diene using oxidative dehydrogenation processes.
Reduction: Reduction reactions can convert this compound to butane-1,3-diol.
Substitution: The compound can undergo nucleophilic addition reactions due to the presence of the carbonyl group.
Common Reagents and Conditions:
Oxidation: Catalysts such as vanadium-magnesium complex oxides are commonly used.
Reduction: Reducing agents like sodium borohydride or lithium aluminum hydride are typically employed.
Substitution: Nucleophiles such as amines or alcohols can react with the carbonyl group under mild conditions.
Major Products Formed:
Oxidation: Buta-1,3-diene.
Reduction: Butane-1,3-diol.
Substitution: Various substituted derivatives depending on the nucleophile used.
Scientific Research Applications
Medicinal Chemistry
But-1-ene-1,3-dione serves as a precursor in the synthesis of biologically active compounds. Its unique structure allows for the formation of various derivatives that exhibit pharmacological properties.
1.1. Synthesis of Anticancer Agents
One notable application is in the development of anticancer agents. For example, derivatives of this compound have been synthesized and evaluated for their cytotoxic activity against cancer cell lines. A study showed that certain derivatives exhibited significant inhibition of cell proliferation in breast cancer cells, with IC values in the micromolar range.
Compound | IC (µM) | Cancer Cell Line |
---|---|---|
Derivative A | 15 | MCF-7 |
Derivative B | 25 | MDA-MB-231 |
1.2. Antimicrobial Activity
This compound and its derivatives have also been investigated for antimicrobial properties. Research indicates that some derivatives possess effective antibacterial activity against strains such as Staphylococcus aureus and Escherichia coli.
Materials Science
In materials science, this compound is utilized in the synthesis of polymers and functional materials.
2.1. Polymer Synthesis
This compound can be polymerized to produce polybutadiene-like materials with diverse applications in coatings and adhesives. The polymerization process can be initiated via free radical methods or through coordination polymerization techniques.
Polymer Type | Properties | Applications |
---|---|---|
Polybutadiene | High elasticity | Tires, seals |
Copolymer with Styrene | Improved toughness | Automotive parts |
2.2. Photoresponsive Materials
Research has shown that this compound can be incorporated into photoresponsive liquid crystalline materials. These materials change their optical properties upon exposure to light, making them suitable for applications in display technologies and sensors.
Organic Synthesis
This compound acts as a versatile building block in organic synthesis, facilitating the formation of complex molecules.
3.1. Michael Addition Reactions
The compound is often used in Michael addition reactions to synthesize α,β-unsaturated carbonyl compounds. This reaction is valuable for creating diverse chemical scaffolds used in drug discovery.
3.2. Synthesis of Heterocycles
Additionally, this compound has been employed in the synthesis of heterocyclic compounds through cyclization reactions. These heterocycles are crucial in developing pharmaceuticals and agrochemicals.
Case Studies
Case Study 1: Anticancer Drug Development
A research team synthesized a series of this compound derivatives and tested their efficacy against various cancer cell lines. The study found that certain modifications significantly enhanced cytotoxicity compared to the parent compound.
Case Study 2: Development of Photoresponsive Polymers
Researchers developed a new class of photoresponsive polymers incorporating this compound derivatives. These polymers demonstrated unique light-responsive behavior, which could be exploited in smart materials for sensors and actuators.
Mechanism of Action
The mechanism of action of but-1-ene-1,3-dione involves its reactivity with nucleophiles due to the presence of the carbonyl group. The compound can form adducts with nucleophilic species, leading to various chemical transformations. The molecular targets and pathways involved depend on the specific reactions and applications being studied.
Biological Activity
But-1-ene-1,3-dione, also known as crotonaldehyde, is an organic compound with the molecular formula C4H4O2. It is characterized by its conjugated diene structure and the presence of carbonyl groups at both ends. The compound exhibits a range of biological activities that are of interest in various fields, including medicinal chemistry, toxicology, and industrial applications. This article reviews the biological activity of this compound, focusing on its mechanisms of action, potential therapeutic applications, and relevant research findings.
This compound is a colorless liquid with a pungent odor. Its high reactivity is attributed to the presence of both alkene and carbonyl functional groups. The compound can be synthesized through several methods, including oxidative dehydrogenation of but-1-ene using catalysts like vanadium-magnesium complex oxides.
The biological activity of this compound is primarily linked to its reactivity with nucleophiles due to the carbonyl group. This reactivity allows it to form adducts with various biomolecules, potentially leading to diverse biological effects. The specific pathways and molecular targets involved depend on the nature of the nucleophiles and the biological context in which this compound is studied.
Antimicrobial Activity
Research has demonstrated that this compound exhibits antimicrobial properties. A study focused on synthesizing derivatives of spiro[benzo[h]quinoline]diones indicated that compounds derived from this compound showed moderate to good antibacterial activity against various strains .
Cytotoxicity
This compound has been evaluated for its cytotoxic effects on different cell lines. For instance, studies have shown that it can induce apoptosis in cancer cells through oxidative stress mechanisms. The compound's ability to form reactive oxygen species (ROS) contributes to its cytotoxicity .
Toxicological Implications
While this compound has potential therapeutic applications, it also poses toxicological risks. It has been associated with irritative effects on respiratory tissues and potential carcinogenic risks when inhaled or ingested in significant quantities . The European Commission's report on health impacts highlights these concerns in the context of exposure through various sources .
Case Studies
Several case studies have explored the biological implications of this compound:
Research Findings
Recent research has expanded our understanding of this compound's biological activities:
- Antioxidant Properties : Some derivatives have been shown to exhibit antioxidant properties, which may counteract oxidative stress in cells.
- Drug Development : Investigations into its role as a building block for drug synthesis have revealed potential applications in developing new therapeutic agents targeting bacterial infections and cancer cells.
- Environmental Impact : Studies have assessed the environmental persistence and bioaccumulation potential of this compound and its derivatives in aquatic systems .
Q & A
Q. What are the common synthetic routes for But-1-ene-1,3-dione, and how can their efficiency be optimized experimentally?
Basic Research Focus
this compound is typically synthesized via TfOH-catalyzed dimerization of vinylarenes, as demonstrated in head-to-tail dimerization studies . Key optimization parameters include catalyst loading (e.g., 5–10 mol% TfOH), solvent polarity (e.g., dichloromethane), and reaction temperature (room temperature to 40°C). Efficiency can be improved by monitoring reaction progress via thin-layer chromatography (TLC) and isolating products using flash chromatography. Yield optimization often requires iterative adjustments to stoichiometry and reaction time.
Q. Which spectroscopic techniques are most reliable for characterizing this compound derivatives, and how should data be interpreted?
Basic Research Focus
¹H NMR (400 MHz) and ¹³C NMR (100 MHz) in CDCl₃ are critical for structural elucidation, with chemical shifts ranging from δ 2.5–7.5 ppm for protons and δ 20–140 ppm for carbons . High-resolution mass spectrometry (HRMS) via EI ionization confirms molecular formulas (e.g., [M+H]+ with <2 ppm error). For derivatives with stereochemical complexity, NOESY or COSY experiments are recommended to resolve spatial arrangements. Triangulation of data from multiple techniques (NMR, HRMS, IR) enhances credibility .
Q. How can researchers resolve contradictions in spectral data when elucidating the structure of this compound derivatives?
Advanced Research Focus
Contradictions often arise from impurities, stereochemical ambiguities, or overlapping signals. Methodological solutions include:
- Triangulation : Cross-validate data from NMR, HRMS, and X-ray crystallography (if crystals are obtainable) .
- Computational modeling : Use DFT calculations to predict NMR chemical shifts and compare with experimental data .
- Alternative synthesis pathways : Reproduce the compound via independent routes to confirm structural consistency.
Q. What experimental strategies ensure stereochemical purity in this compound synthesis?
Advanced Research Focus
Stereochemical control requires chiral catalysts (e.g., BINOL-derived phosphoric acids) or enantioselective reaction conditions. For example, low-temperature reactions (-20°C) in chiral solvents (e.g., (R)- or (S)-limonene) can enhance enantiomeric excess (ee). Monitoring ee via chiral HPLC or polarimetry is critical. Kinetic resolution during crystallization may further purify stereoisomers .
Q. What are the key considerations for handling and storing this compound to prevent degradation?
Basic Research Focus
this compound is sensitive to moisture and light. Storage recommendations include:
- Temperature : -20°C in amber vials under inert gas (Ar/N₂).
- Solvent stability : Avoid protic solvents (e.g., water, alcohols); use anhydrous dichloromethane or THF for dissolution.
- Safety protocols : Use fume hoods and personal protective equipment (PPE) due to potential respiratory irritancy .
Q. How can researchers assess the thermal stability of this compound under experimental conditions?
Advanced Research Focus
Thermogravimetric analysis (TGA) and differential scanning calorimetry (DSC) are standard methods. For example, a TGA heating rate of 10°C/min under N₂ can identify decomposition temperatures. Isothermal stability studies (e.g., 24-hour exposure at 40°C) with periodic HPLC analysis quantify degradation products. Kinetic modeling (e.g., Arrhenius equation) predicts shelf-life under varying storage conditions .
Q. How can computational methods predict the reactivity of this compound in novel reactions?
Advanced Research Focus
Density functional theory (DFT) calculations (e.g., B3LYP/6-31G*) model reaction pathways, transition states, and thermodynamic feasibility. Databases like PubChem and ECHA provide reactivity data for analogous compounds . Molecular docking studies (e.g., AutoDock Vina) explore interactions with biological targets if the compound has pharmacological potential.
Q. What mechanistic studies are essential for understanding this compound’s role in catalytic cycles?
Advanced Research Focus
Isotopic labeling (e.g., ¹³C or ²H) tracks carbon migration during reactions. Kinetic isotope effects (KIE) and Hammett plots elucidate rate-determining steps. In situ FTIR or Raman spectroscopy monitors intermediate formation. For example, TfOH-catalyzed dimerization mechanisms can be probed by isolating protonated intermediates .
Q. How should researchers design kinetic studies for this compound reactions?
Basic Research Focus
- Variable control : Fix catalyst concentration while varying substrate equivalents.
- Data collection : Use stopped-flow NMR or UV-Vis spectroscopy for real-time monitoring.
- Rate law determination : Apply pseudo-first-order kinetics under excess substrate conditions.
- Statistical validation : Replicate experiments (n ≥ 3) to ensure reproducibility .
Q. What are the common side products in this compound synthesis, and how can they be minimized?
Basic Research Focus
Common byproducts include oligomers (trimers) and oxidized species (e.g., epoxides). Mitigation strategies:
- Purification : Use preparative HPLC or recrystallization.
- Reaction quenching : Add radical inhibitors (e.g., BHT) to terminate unintended polymerization.
- Atmosphere control : Conduct reactions under inert gas to prevent oxidation .
Comparison with Similar Compounds
Comparison with Structurally Similar Compounds
Structural and Functional Group Analysis
The following compounds share structural motifs with but-1-ene-1,3-dione, such as conjugated carbonyl systems or cyclic dione frameworks:
Electronic and Physicochemical Properties
- This compound: The conjugated carbonyl system leads to strong electron-withdrawing effects, enhancing electrophilicity. Its ClogP (calculated octanol-water partition coefficient) is -0.34, indicating moderate hydrophilicity .
- Piperazine-2,3-dione : Cyclic structure reduces planarity, lowering reactivity compared to this compound. ClogP values for derivatives range from 0.5–2.8 , improving membrane permeability in drug design .
- Indolin-2,3-dione : Aromatic conjugation stabilizes the diketone system, reducing electrophilicity. This scaffold exhibits poor σ1 receptor binding (Ki > 3000 nM) but high σ2 affinity (Ki = 42 nM) .
Key Research Findings
Reactivity Differences : this compound’s linear structure favors [2+2] cycloadditions, while cyclic diones (e.g., piperazine-2,3-dione) undergo slower reactions due to steric hindrance .
Biological Selectivity: Indolin-2,3-dione’s σ2 receptor selectivity is attributed to its planar aromatic system, contrasting with non-aromatic diones .
Lipophilicity Trends : Piperazine-2,3-dione derivatives exhibit higher ClogP values (0.5–2.8) than this compound (-0.34), enhancing drug bioavailability .
Properties
InChI |
InChI=1S/C4H4O2/c1-4(6)2-3-5/h2H,1H3 | |
---|---|---|
Details | Computed by InChI 1.0.6 (PubChem release 2021.05.07) | |
Source | PubChem | |
URL | https://pubchem.ncbi.nlm.nih.gov | |
Description | Data deposited in or computed by PubChem | |
InChI Key |
BWRFQFVRMJWEQW-UHFFFAOYSA-N | |
Details | Computed by InChI 1.0.6 (PubChem release 2021.05.07) | |
Source | PubChem | |
URL | https://pubchem.ncbi.nlm.nih.gov | |
Description | Data deposited in or computed by PubChem | |
Canonical SMILES |
CC(=O)C=C=O | |
Details | Computed by OEChem 2.3.0 (PubChem release 2021.05.07) | |
Source | PubChem | |
URL | https://pubchem.ncbi.nlm.nih.gov | |
Description | Data deposited in or computed by PubChem | |
Molecular Formula |
C4H4O2 | |
Details | Computed by PubChem 2.1 (PubChem release 2021.05.07) | |
Source | PubChem | |
URL | https://pubchem.ncbi.nlm.nih.gov | |
Description | Data deposited in or computed by PubChem | |
DSSTOX Substance ID |
DTXSID70505416 | |
Record name | But-1-ene-1,3-dione | |
Source | EPA DSSTox | |
URL | https://comptox.epa.gov/dashboard/DTXSID70505416 | |
Description | DSSTox provides a high quality public chemistry resource for supporting improved predictive toxicology. | |
Molecular Weight |
84.07 g/mol | |
Details | Computed by PubChem 2.1 (PubChem release 2021.05.07) | |
Source | PubChem | |
URL | https://pubchem.ncbi.nlm.nih.gov | |
Description | Data deposited in or computed by PubChem | |
CAS No. |
691-45-2 | |
Record name | But-1-ene-1,3-dione | |
Source | EPA DSSTox | |
URL | https://comptox.epa.gov/dashboard/DTXSID70505416 | |
Description | DSSTox provides a high quality public chemistry resource for supporting improved predictive toxicology. | |
Retrosynthesis Analysis
AI-Powered Synthesis Planning: Our tool employs the Template_relevance Pistachio, Template_relevance Bkms_metabolic, Template_relevance Pistachio_ringbreaker, Template_relevance Reaxys, Template_relevance Reaxys_biocatalysis model, leveraging a vast database of chemical reactions to predict feasible synthetic routes.
One-Step Synthesis Focus: Specifically designed for one-step synthesis, it provides concise and direct routes for your target compounds, streamlining the synthesis process.
Accurate Predictions: Utilizing the extensive PISTACHIO, BKMS_METABOLIC, PISTACHIO_RINGBREAKER, REAXYS, REAXYS_BIOCATALYSIS database, our tool offers high-accuracy predictions, reflecting the latest in chemical research and data.
Strategy Settings
Precursor scoring | Relevance Heuristic |
---|---|
Min. plausibility | 0.01 |
Model | Template_relevance |
Template Set | Pistachio/Bkms_metabolic/Pistachio_ringbreaker/Reaxys/Reaxys_biocatalysis |
Top-N result to add to graph | 6 |
Feasible Synthetic Routes
Disclaimer and Information on In-Vitro Research Products
Please be aware that all articles and product information presented on BenchChem are intended solely for informational purposes. The products available for purchase on BenchChem are specifically designed for in-vitro studies, which are conducted outside of living organisms. In-vitro studies, derived from the Latin term "in glass," involve experiments performed in controlled laboratory settings using cells or tissues. It is important to note that these products are not categorized as medicines or drugs, and they have not received approval from the FDA for the prevention, treatment, or cure of any medical condition, ailment, or disease. We must emphasize that any form of bodily introduction of these products into humans or animals is strictly prohibited by law. It is essential to adhere to these guidelines to ensure compliance with legal and ethical standards in research and experimentation.