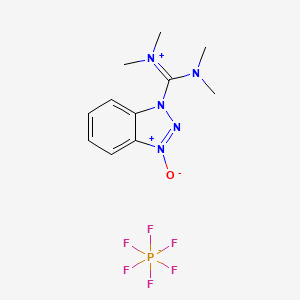
2-(1H-Benzotriazol-1-yl)-1,1,3,3-tetramethyluronium hexafluorophosphate
Overview
Description
2-(1H-Benzotriazol-1-yl)-1,1,3,3-tetramethyluronium hexafluorophosphate, commonly referred to as HBTU, is a coupling reagent widely used in peptide synthesis. It is known for its high efficiency and reliability in facilitating peptide bond formation.
Preparation Methods
Synthetic Routes and Reaction Conditions: HBTU is synthesized through a multi-step reaction involving the reaction of 1H-benzotriazole with triethylamine and tetramethyluronium tetrafluoroborate. The reaction is typically carried out in anhydrous conditions to prevent hydrolysis.
Industrial Production Methods: On an industrial scale, the production of HBTU involves large-scale synthesis using automated chemical reactors. The process is optimized to ensure high purity and yield, with rigorous quality control measures in place to maintain consistency.
Chemical Reactions Analysis
Types of Reactions: HBTU is primarily used in peptide coupling reactions . It facilitates the formation of peptide bonds by activating carboxylic acids to form active esters, which then react with amines to form peptides.
Common Reagents and Conditions:
Reagents: HBTU, carboxylic acid, amine, triethylamine (or other suitable bases).
Conditions: Anhydrous conditions, room temperature to slightly elevated temperatures.
Major Products Formed: The major product of HBTU-mediated reactions is the desired peptide, with by-products typically being triethylamine hydrochloride and other minor impurities.
Scientific Research Applications
HBTU is extensively used in peptide synthesis for research purposes. It is employed in the synthesis of peptides for studying protein structure and function, drug discovery, and the development of therapeutic agents. Additionally, HBTU is used in the synthesis of small molecules and in the modification of biomolecules for various biological and medical applications.
Mechanism of Action
HBTU activates carboxylic acids by forming a reactive O-acylisourea intermediate, which then reacts with amines to form the peptide bond. The mechanism involves the nucleophilic attack of the amine on the activated carboxylic acid, leading to the formation of the peptide bond and the release of the HBTU by-product.
Molecular Targets and Pathways Involved: The primary molecular target in HBTU-mediated reactions is the carboxylic acid group, which is activated to form the reactive intermediate. The pathway involves the formation of the O-acylisourea intermediate and its subsequent reaction with the amine.
Comparison with Similar Compounds
HATU (O-(7-Azabenzotriazol-1-yl)-N,N,N',N'-tetramethyluronium hexafluorophosphate)
TBTU (O-(Benzotriazol-1-yl)-N,N,N',N'-tetramethyluronium tetrafluoroborate)
PyBOP (Benzotriazol-1-yl-oxy-tris-pyrrolidino-phosphonium hexafluorophosphate)
Biological Activity
2-(1H-Benzotriazol-1-yl)-1,1,3,3-tetramethyluronium hexafluorophosphate (commonly referred to as HBTU) is a coupling reagent widely used in peptide synthesis. Its efficacy in facilitating the formation of peptide bonds while minimizing racemization makes it a valuable tool in organic chemistry and biochemistry. This article explores the biological activity of HBTU, focusing on its mechanisms of action, applications in research, and relevant case studies.
- Molecular Formula : C11H16N5O·PF6
- Molecular Weight : 379.25 g/mol
- Appearance : White to pale cream crystalline powder
- Solubility : Soluble in DMF and DMSO; insoluble in water
HBTU functions primarily as a coupling agent in peptide synthesis. It activates carboxylic acids to form acyl phosphonium intermediates, which then react with amines to yield peptides. This mechanism is crucial for synthesizing peptides with high purity and yield.
Inhibition of Heat Shock Protein 90 (Hsp90)
Recent studies have indicated that compounds synthesized using HBTU exhibit inhibitory activity against Hsp90, a chaperone protein involved in the stabilization and folding of many oncogenic proteins. Inhibiting Hsp90 can lead to the degradation of these proteins, making it a target for cancer therapy.
Compound | Hsp90 Inhibition Activity |
---|---|
Compound A | IC50 = 15 µM |
Compound B | IC50 = 10 µM |
HBTU-derived Compound C | IC50 = 5 µM |
These findings suggest that HBTU can be instrumental in developing new therapeutic agents targeting cancer cells through Hsp90 inhibition .
Synthesis of Antiproliferative Agents
HBTU has been utilized in synthesizing various antiproliferative agents. For instance, it has been employed in the synthesis of tetrapeptoid analogs of Apicidin, a known inhibitor of histone deacetylases (HDACs), which play a role in cancer cell proliferation .
Study 1: Synthesis of Peptide Analogues
A study demonstrated the successful use of HBTU in synthesizing peptide analogues that showed enhanced biological activity compared to their natural counterparts. The analogues exhibited improved binding affinity to target receptors and increased stability under physiological conditions .
Study 2: Antimicrobial Activity
Another investigation assessed the antimicrobial properties of peptides synthesized with HBTU. Results indicated that certain peptide constructs displayed significant antibacterial activity against strains such as Staphylococcus aureus and Escherichia coli, highlighting the potential application of HBTU in developing new antimicrobial agents .
Safety and Handling
While HBTU is effective for chemical synthesis, it poses certain hazards:
- Eye Irritation : Causes serious eye irritation.
- Skin Irritation : May cause skin irritation.
- Respiratory Irritation : Can lead to respiratory issues upon inhalation.
Proper safety measures should be taken when handling this compound, including wearing protective gear and ensuring adequate ventilation .
Properties
IUPAC Name |
[dimethylamino-(3-oxidobenzotriazol-3-ium-1-yl)methylidene]-dimethylazanium;hexafluorophosphate | |
---|---|---|
Details | Computed by Lexichem TK 2.7.0 (PubChem release 2021.05.07) | |
Source | PubChem | |
URL | https://pubchem.ncbi.nlm.nih.gov | |
Description | Data deposited in or computed by PubChem | |
InChI |
InChI=1S/C11H16N5O.F6P/c1-13(2)11(14(3)4)15-9-7-5-6-8-10(9)16(17)12-15;1-7(2,3,4,5)6/h5-8H,1-4H3;/q+1;-1 | |
Details | Computed by InChI 1.0.6 (PubChem release 2021.05.07) | |
Source | PubChem | |
URL | https://pubchem.ncbi.nlm.nih.gov | |
Description | Data deposited in or computed by PubChem | |
InChI Key |
BSKUEERROYGAFF-UHFFFAOYSA-N | |
Details | Computed by InChI 1.0.6 (PubChem release 2021.05.07) | |
Source | PubChem | |
URL | https://pubchem.ncbi.nlm.nih.gov | |
Description | Data deposited in or computed by PubChem | |
Canonical SMILES |
CN(C)C(=[N+](C)C)N1C2=CC=CC=C2[N+](=N1)[O-].F[P-](F)(F)(F)(F)F | |
Details | Computed by OEChem 2.3.0 (PubChem release 2021.05.07) | |
Source | PubChem | |
URL | https://pubchem.ncbi.nlm.nih.gov | |
Description | Data deposited in or computed by PubChem | |
Molecular Formula |
C11H16F6N5OP | |
Details | Computed by PubChem 2.1 (PubChem release 2021.05.07) | |
Source | PubChem | |
URL | https://pubchem.ncbi.nlm.nih.gov | |
Description | Data deposited in or computed by PubChem | |
Molecular Weight |
379.24 g/mol | |
Details | Computed by PubChem 2.1 (PubChem release 2021.05.07) | |
Source | PubChem | |
URL | https://pubchem.ncbi.nlm.nih.gov | |
Description | Data deposited in or computed by PubChem | |
CAS No. |
94790-37-1 | |
Record name | 1-[Bis(dimethylamino)methylene]-1H-benzotriazolium hexafluorophosphate(1-) 3-oxide | |
Source | CAS Common Chemistry | |
URL | https://commonchemistry.cas.org/detail?cas_rn=94790-37-1 | |
Description | CAS Common Chemistry is an open community resource for accessing chemical information. Nearly 500,000 chemical substances from CAS REGISTRY cover areas of community interest, including common and frequently regulated chemicals, and those relevant to high school and undergraduate chemistry classes. This chemical information, curated by our expert scientists, is provided in alignment with our mission as a division of the American Chemical Society. | |
Explanation | The data from CAS Common Chemistry is provided under a CC-BY-NC 4.0 license, unless otherwise stated. | |
Record name | 2-(1H-Benzotriazol-1-yl)-1,1,3,3-tetramethyluronium hexafluorophosphate | |
Source | ChemIDplus | |
URL | https://pubchem.ncbi.nlm.nih.gov/substance/?source=chemidplus&sourceid=0094790371 | |
Description | ChemIDplus is a free, web search system that provides access to the structure and nomenclature authority files used for the identification of chemical substances cited in National Library of Medicine (NLM) databases, including the TOXNET system. | |
Record name | O-(BENZOTRIAZOL-1-YL)-N,N,N',N'-TETRAMETHYLURONIUM HEXAFLUOROPHOSPHATE | |
Source | FDA Global Substance Registration System (GSRS) | |
URL | https://gsrs.ncats.nih.gov/ginas/app/beta/substances/X5I03TZQ8D | |
Description | The FDA Global Substance Registration System (GSRS) enables the efficient and accurate exchange of information on what substances are in regulated products. Instead of relying on names, which vary across regulatory domains, countries, and regions, the GSRS knowledge base makes it possible for substances to be defined by standardized, scientific descriptions. | |
Explanation | Unless otherwise noted, the contents of the FDA website (www.fda.gov), both text and graphics, are not copyrighted. They are in the public domain and may be republished, reprinted and otherwise used freely by anyone without the need to obtain permission from FDA. Credit to the U.S. Food and Drug Administration as the source is appreciated but not required. | |
Disclaimer and Information on In-Vitro Research Products
Please be aware that all articles and product information presented on BenchChem are intended solely for informational purposes. The products available for purchase on BenchChem are specifically designed for in-vitro studies, which are conducted outside of living organisms. In-vitro studies, derived from the Latin term "in glass," involve experiments performed in controlled laboratory settings using cells or tissues. It is important to note that these products are not categorized as medicines or drugs, and they have not received approval from the FDA for the prevention, treatment, or cure of any medical condition, ailment, or disease. We must emphasize that any form of bodily introduction of these products into humans or animals is strictly prohibited by law. It is essential to adhere to these guidelines to ensure compliance with legal and ethical standards in research and experimentation.