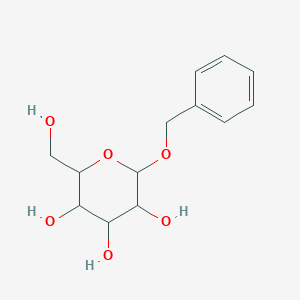
2-(Hydroxymethyl)-6-phenylmethoxyoxane-3,4,5-triol
Overview
Description
2-(Hydroxymethyl)-6-phenylmethoxyoxane-3,4,5-triol is a complex organic compound characterized by its multiple hydroxyl groups and phenylmethoxy group
Preparation Methods
Synthetic Routes and Reaction Conditions: The synthesis of 2-(Hydroxymethyl)-6-phenylmethoxyoxane-3,4,5-triol typically involves multiple steps, starting with the appropriate phenylmethoxy precursor. The hydroxymethyl group is introduced through a hydroxymethylation reaction, often using formaldehyde in a basic medium. Subsequent steps may include oxidation and protection/deprotection of hydroxyl groups to achieve the desired structure.
Industrial Production Methods: On an industrial scale, the production of this compound would require optimized reaction conditions to ensure high yield and purity. Continuous flow reactors and advanced purification techniques, such as column chromatography, may be employed to streamline the process.
Chemical Reactions Analysis
Types of Reactions: This compound can undergo various chemical reactions, including:
Oxidation: The hydroxyl groups can be oxidized to form carbonyl compounds.
Reduction: Reduction reactions can be used to convert carbonyl groups back to hydroxyl groups.
Substitution: The phenylmethoxy group can participate in electrophilic aromatic substitution reactions.
Common Reagents and Conditions:
Oxidation: Common oxidizing agents include chromium(VI) oxide and Dess-Martin periodinane.
Reduction: Reducing agents like lithium aluminum hydride (LiAlH4) are often used.
Substitution: Electrophilic substitution typically requires strong acids or Lewis acids.
Major Products Formed:
Oxidation: Formation of aldehydes or ketones.
Reduction: Formation of alcohols.
Substitution: Introduction of new substituents on the aromatic ring.
Scientific Research Applications
Chemistry: In chemistry, this compound can serve as a versatile intermediate for synthesizing more complex molecules. Its multiple hydroxyl groups make it a potential candidate for further functionalization.
Biology: In biological research, 2-(Hydroxymethyl)-6-phenylmethoxyoxane-3,4,5-triol may be used to study enzyme-substrate interactions or as a building block for bioactive molecules.
Medicine: Potential medicinal applications include the development of new drugs or therapeutic agents. Its structural complexity allows for interactions with various biological targets.
Industry: In the industrial sector, this compound could be used in the production of advanced materials, such as polymers or coatings, due to its functional groups.
Mechanism of Action
The mechanism by which 2-(Hydroxymethyl)-6-phenylmethoxyoxane-3,4,5-triol exerts its effects depends on its specific application. For example, in medicinal chemistry, it may interact with enzymes or receptors through hydrogen bonding or hydrophobic interactions. The molecular targets and pathways involved would vary based on the biological context.
Comparison with Similar Compounds
2-(Hydroxymethyl)-6-methoxyoxane-3,4,5-triol: Similar structure but with a methoxy group instead of phenylmethoxy.
2-(Hydroxymethyl)-6-phenylmethoxyoxane-2,3,4-triol: Different position of hydroxyl groups.
Uniqueness: The presence of the phenylmethoxy group in 2-(Hydroxymethyl)-6-phenylmethoxyoxane-3,4,5-triol provides unique chemical properties compared to its analogs. This group can influence the compound's reactivity and interaction with biological targets.
Biological Activity
2-(Hydroxymethyl)-6-phenylmethoxyoxane-3,4,5-triol, also referred to by its IUPAC name and CAS number (34246-23-6), is a compound of significant interest in biochemical and pharmaceutical research. This article explores its biological activity, mechanisms of action, and potential therapeutic applications based on diverse sources.
Chemical Structure and Properties
The molecular formula of this compound is with a molecular weight of approximately 270.278 g/mol. The compound features several hydroxymethyl groups and a phenylmethoxy group, which contribute to its reactivity and biological interactions.
Property | Value |
---|---|
Molecular Formula | |
Molecular Weight | 270.278 g/mol |
Melting Point | 111-112 °C |
The biological activity of this compound is largely attributed to its ability to interact with various biomolecules. It acts as a glycosyl acceptor in enzymatic reactions, facilitating the formation of glycosidic bonds that are crucial for oligosaccharide synthesis. The presence of hydroxyl groups allows it to participate in hydrogen bonding and other interactions that enhance its solubility and bioavailability.
Interaction with Biological Targets
- Enzyme Inhibition : Studies indicate that this compound can inhibit certain glycosidases, thereby modulating carbohydrate metabolism.
- Cell Signaling : The compound may influence cell signaling pathways through its interaction with lectins, promoting or inhibiting cellular adhesion processes critical for various biological functions.
Antimicrobial Properties
Research has shown that derivatives of this compound exhibit antimicrobial activity against specific bacterial strains. For instance, it has been noted to prevent bacterial adhesion by acting as an antagonist to FimH, a protein involved in the adhesion of bacteria to host cells.
Antitumor Activity
In animal models, higher doses of this compound have been associated with significant tumor-inhibiting effects. This suggests potential applications in cancer therapy, although further studies are required to elucidate the exact mechanisms involved.
Case Studies
- Case Study on Glycosylation : A study demonstrated the use of this compound in synthesizing complex oligosaccharides. The ability to act as a glycosyl acceptor allowed researchers to create novel carbohydrate structures that could be utilized in drug development.
- Clinical Applications : In clinical settings, compounds similar to this compound have been explored for their potential in treating metabolic disorders like type 2 diabetes due to their effects on glucose metabolism and insulin sensitivity .
Properties
IUPAC Name |
2-(hydroxymethyl)-6-phenylmethoxyoxane-3,4,5-triol | |
---|---|---|
Details | Computed by LexiChem 2.6.6 (PubChem release 2019.06.18) | |
Source | PubChem | |
URL | https://pubchem.ncbi.nlm.nih.gov | |
Description | Data deposited in or computed by PubChem | |
InChI |
InChI=1S/C13H18O6/c14-6-9-10(15)11(16)12(17)13(19-9)18-7-8-4-2-1-3-5-8/h1-5,9-17H,6-7H2 | |
Details | Computed by InChI 1.0.5 (PubChem release 2019.06.18) | |
Source | PubChem | |
URL | https://pubchem.ncbi.nlm.nih.gov | |
Description | Data deposited in or computed by PubChem | |
InChI Key |
GKHCBYYBLTXYEV-UHFFFAOYSA-N | |
Details | Computed by InChI 1.0.5 (PubChem release 2019.06.18) | |
Source | PubChem | |
URL | https://pubchem.ncbi.nlm.nih.gov | |
Description | Data deposited in or computed by PubChem | |
Canonical SMILES |
C1=CC=C(C=C1)COC2C(C(C(C(O2)CO)O)O)O | |
Details | Computed by OEChem 2.1.5 (PubChem release 2019.06.18) | |
Source | PubChem | |
URL | https://pubchem.ncbi.nlm.nih.gov | |
Description | Data deposited in or computed by PubChem | |
Molecular Formula |
C13H18O6 | |
Details | Computed by PubChem 2.1 (PubChem release 2019.06.18) | |
Source | PubChem | |
URL | https://pubchem.ncbi.nlm.nih.gov | |
Description | Data deposited in or computed by PubChem | |
DSSTOX Substance ID |
DTXSID40407251 | |
Record name | 2-(hydroxymethyl)-6-phenylmethoxyoxane-3,4,5-triol | |
Source | EPA DSSTox | |
URL | https://comptox.epa.gov/dashboard/DTXSID40407251 | |
Description | DSSTox provides a high quality public chemistry resource for supporting improved predictive toxicology. | |
Molecular Weight |
270.28 g/mol | |
Details | Computed by PubChem 2.1 (PubChem release 2021.05.07) | |
Source | PubChem | |
URL | https://pubchem.ncbi.nlm.nih.gov | |
Description | Data deposited in or computed by PubChem | |
CAS No. |
15548-45-5 | |
Record name | 2-(hydroxymethyl)-6-phenylmethoxyoxane-3,4,5-triol | |
Source | EPA DSSTox | |
URL | https://comptox.epa.gov/dashboard/DTXSID40407251 | |
Description | DSSTox provides a high quality public chemistry resource for supporting improved predictive toxicology. | |
Synthesis routes and methods
Procedure details
Retrosynthesis Analysis
AI-Powered Synthesis Planning: Our tool employs the Template_relevance Pistachio, Template_relevance Bkms_metabolic, Template_relevance Pistachio_ringbreaker, Template_relevance Reaxys, Template_relevance Reaxys_biocatalysis model, leveraging a vast database of chemical reactions to predict feasible synthetic routes.
One-Step Synthesis Focus: Specifically designed for one-step synthesis, it provides concise and direct routes for your target compounds, streamlining the synthesis process.
Accurate Predictions: Utilizing the extensive PISTACHIO, BKMS_METABOLIC, PISTACHIO_RINGBREAKER, REAXYS, REAXYS_BIOCATALYSIS database, our tool offers high-accuracy predictions, reflecting the latest in chemical research and data.
Strategy Settings
Precursor scoring | Relevance Heuristic |
---|---|
Min. plausibility | 0.01 |
Model | Template_relevance |
Template Set | Pistachio/Bkms_metabolic/Pistachio_ringbreaker/Reaxys/Reaxys_biocatalysis |
Top-N result to add to graph | 6 |
Feasible Synthetic Routes
Disclaimer and Information on In-Vitro Research Products
Please be aware that all articles and product information presented on BenchChem are intended solely for informational purposes. The products available for purchase on BenchChem are specifically designed for in-vitro studies, which are conducted outside of living organisms. In-vitro studies, derived from the Latin term "in glass," involve experiments performed in controlled laboratory settings using cells or tissues. It is important to note that these products are not categorized as medicines or drugs, and they have not received approval from the FDA for the prevention, treatment, or cure of any medical condition, ailment, or disease. We must emphasize that any form of bodily introduction of these products into humans or animals is strictly prohibited by law. It is essential to adhere to these guidelines to ensure compliance with legal and ethical standards in research and experimentation.