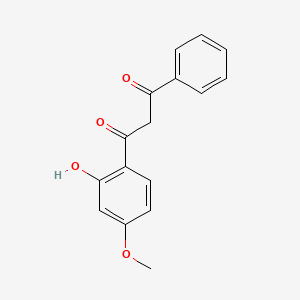
1-(2-Hydroxy-4-methoxyphenyl)-3-phenylpropane-1,3-dione
Overview
Description
1-(2-Hydroxy-4-methoxyphenyl)-3-phenylpropane-1,3-dione is an organic compound with a complex structure that includes both hydroxy and methoxy functional groups
Preparation Methods
Synthetic Routes and Reaction Conditions
The synthesis of 1-(2-Hydroxy-4-methoxyphenyl)-3-phenylpropane-1,3-dione typically involves the condensation of appropriate starting materials under controlled conditions. One common method involves the use of 2-hydroxy-4-methoxybenzaldehyde and acetophenone in the presence of a base such as sodium hydroxide. The reaction is carried out in an organic solvent like ethanol, and the mixture is refluxed for several hours to yield the desired product .
Industrial Production Methods
On an industrial scale, the production of this compound may involve more efficient and scalable methods. These could include the use of continuous flow reactors and optimized reaction conditions to maximize yield and purity. The use of catalysts and advanced purification techniques such as recrystallization and chromatography may also be employed to ensure the quality of the final product .
Chemical Reactions Analysis
Types of Reactions
1-(2-Hydroxy-4-methoxyphenyl)-3-phenylpropane-1,3-dione can undergo various chemical reactions, including:
Oxidation: This compound can be oxidized to form quinones or other oxidized derivatives.
Reduction: Reduction reactions can convert the carbonyl groups to alcohols.
Substitution: The hydroxy and methoxy groups can participate in nucleophilic substitution reactions.
Common Reagents and Conditions
Oxidation: Common oxidizing agents include potassium permanganate (KMnO₄) and chromium trioxide (CrO₃).
Reduction: Reducing agents such as sodium borohydride (NaBH₄) or lithium aluminum hydride (LiAlH₄) are typically used.
Substitution: Reagents like alkyl halides or acyl chlorides can be used for substitution reactions under basic or acidic conditions.
Major Products
The major products formed from these reactions depend on the specific conditions and reagents used. For example, oxidation may yield quinones, while reduction can produce alcohols. Substitution reactions can introduce various functional groups, leading to a wide range of derivatives .
Scientific Research Applications
1-(2-Hydroxy-4-methoxyphenyl)-3-phenylpropane-1,3-dione has several applications in scientific research:
Chemistry: It is used as a building block for the synthesis of more complex molecules and as a reagent in various organic reactions.
Biology: The compound has been studied for its potential biological activities, including antimicrobial and antioxidant properties.
Medicine: Research has explored its potential therapeutic effects, such as anti-inflammatory and analgesic activities.
Industry: It is used in the production of pharmaceuticals, agrochemicals, and other fine chemicals.
Mechanism of Action
The mechanism of action of 1-(2-Hydroxy-4-methoxyphenyl)-3-phenylpropane-1,3-dione involves its interaction with various molecular targets and pathways. The hydroxy and methoxy groups can participate in hydrogen bonding and other interactions with biological molecules. These interactions can modulate the activity of enzymes, receptors, and other proteins, leading to the observed biological effects .
Comparison with Similar Compounds
Similar Compounds
2-Hydroxy-4-methoxyacetophenone: This compound shares the hydroxy and methoxy functional groups but has a simpler structure.
Paeonol: Known for its biological activities, paeonol has a similar phenolic structure.
2-Hydroxy-4-methoxybenzaldehyde: Another related compound with similar functional groups but different reactivity.
Uniqueness
1-(2-Hydroxy-4-methoxyphenyl)-3-phenylpropane-1,3-dione is unique due to its specific combination of functional groups and its ability to undergo a wide range of chemical reactions. Its structure allows for diverse applications in various fields, making it a valuable compound for research and industrial purposes .
Properties
IUPAC Name |
1-(2-hydroxy-4-methoxyphenyl)-3-phenylpropane-1,3-dione | |
---|---|---|
Details | Computed by Lexichem TK 2.7.0 (PubChem release 2021.05.07) | |
Source | PubChem | |
URL | https://pubchem.ncbi.nlm.nih.gov | |
Description | Data deposited in or computed by PubChem | |
InChI |
InChI=1S/C16H14O4/c1-20-12-7-8-13(15(18)9-12)16(19)10-14(17)11-5-3-2-4-6-11/h2-9,18H,10H2,1H3 | |
Details | Computed by InChI 1.0.6 (PubChem release 2021.05.07) | |
Source | PubChem | |
URL | https://pubchem.ncbi.nlm.nih.gov | |
Description | Data deposited in or computed by PubChem | |
InChI Key |
JZKDEMPGCWWFCP-UHFFFAOYSA-N | |
Details | Computed by InChI 1.0.6 (PubChem release 2021.05.07) | |
Source | PubChem | |
URL | https://pubchem.ncbi.nlm.nih.gov | |
Description | Data deposited in or computed by PubChem | |
Canonical SMILES |
COC1=CC(=C(C=C1)C(=O)CC(=O)C2=CC=CC=C2)O | |
Details | Computed by OEChem 2.3.0 (PubChem release 2021.05.07) | |
Source | PubChem | |
URL | https://pubchem.ncbi.nlm.nih.gov | |
Description | Data deposited in or computed by PubChem | |
Molecular Formula |
C16H14O4 | |
Details | Computed by PubChem 2.1 (PubChem release 2021.05.07) | |
Source | PubChem | |
URL | https://pubchem.ncbi.nlm.nih.gov | |
Description | Data deposited in or computed by PubChem | |
Molecular Weight |
270.28 g/mol | |
Details | Computed by PubChem 2.1 (PubChem release 2021.05.07) | |
Source | PubChem | |
URL | https://pubchem.ncbi.nlm.nih.gov | |
Description | Data deposited in or computed by PubChem | |
Retrosynthesis Analysis
AI-Powered Synthesis Planning: Our tool employs the Template_relevance Pistachio, Template_relevance Bkms_metabolic, Template_relevance Pistachio_ringbreaker, Template_relevance Reaxys, Template_relevance Reaxys_biocatalysis model, leveraging a vast database of chemical reactions to predict feasible synthetic routes.
One-Step Synthesis Focus: Specifically designed for one-step synthesis, it provides concise and direct routes for your target compounds, streamlining the synthesis process.
Accurate Predictions: Utilizing the extensive PISTACHIO, BKMS_METABOLIC, PISTACHIO_RINGBREAKER, REAXYS, REAXYS_BIOCATALYSIS database, our tool offers high-accuracy predictions, reflecting the latest in chemical research and data.
Strategy Settings
Precursor scoring | Relevance Heuristic |
---|---|
Min. plausibility | 0.01 |
Model | Template_relevance |
Template Set | Pistachio/Bkms_metabolic/Pistachio_ringbreaker/Reaxys/Reaxys_biocatalysis |
Top-N result to add to graph | 6 |
Feasible Synthetic Routes
Disclaimer and Information on In-Vitro Research Products
Please be aware that all articles and product information presented on BenchChem are intended solely for informational purposes. The products available for purchase on BenchChem are specifically designed for in-vitro studies, which are conducted outside of living organisms. In-vitro studies, derived from the Latin term "in glass," involve experiments performed in controlled laboratory settings using cells or tissues. It is important to note that these products are not categorized as medicines or drugs, and they have not received approval from the FDA for the prevention, treatment, or cure of any medical condition, ailment, or disease. We must emphasize that any form of bodily introduction of these products into humans or animals is strictly prohibited by law. It is essential to adhere to these guidelines to ensure compliance with legal and ethical standards in research and experimentation.