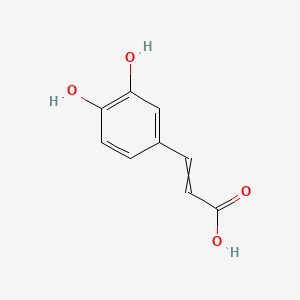
caffeic acid
Overview
Description
“3-(3,4-Dihydroxyphenyl)prop-2-enoic acid”, also known as caffeic acid, is a naturally occurring hydroxycinnamic acid with a variety of biological activities such as antioxidant, anti-inflammatory, antimicrobial, and cytostatic . It is widely distributed in many kinds of traditional Chinese medicine plants such as artemisia, thistle, honeysuckle, etc .
Molecular Structure Analysis
The molecular formula of “3-(3,4-Dihydroxyphenyl)prop-2-enoic acid” is C9H8O4, and its molecular weight is 180.16 . The structure of the compound was confirmed by 1H NMR and 13C NMR spectrum .
Physical and Chemical Properties Analysis
“3-(3,4-Dihydroxyphenyl)prop-2-enoic acid” appears as yellow crystals. It has a melting point of 211-213 °C (dec.) (lit.) and a boiling point of 272.96°C (rough estimate). It is soluble in hot water and slightly soluble in cold water, easily soluble in hot water and cold ethanol .
Scientific Research Applications
1. Molecular Structure Analysis
3-(3,4-Dihydroxyphenyl)prop-2-enoic acid has been explored for its molecular structure and interactions. The hydrogen-bonded chains and weak intermolecular interactions play a key role in its three-dimensional network structure (Yang et al., 2006).
2. Crystallography and Spectroscopy
Structural investigations using X-ray crystallography and spectroscopy have been conducted to understand the compound's characteristics better. These studies provide insights into the compound's stability and molecular interactions (Venkatesan et al., 2016).
3. Radical-Scavenging Activity
The compound has been studied for its nitrogen dioxide radical-scavenging activity. This research is crucial for understanding its potential as an antioxidant and its effectiveness compared to other similar compounds (Kong et al., 2004).
4. Synthesis and Application in Materials Science
Synthetic approaches to modify 3-(3,4-Dihydroxyphenyl)prop-2-enoic acid and its derivatives have been explored for applications in materials science, such as in liquid crystal display technology (Hegde et al., 2013).
5. Catalytic Applications
The compound has been used in palladium-catalysed cross-coupling reactions, showcasing its potential in synthetic chemistry and catalysis (Abarbri et al., 2002).
6. Antioxidant Properties
Studies have highlighted its potential as an antioxidant. This includes investigations into its scavenging abilities and effectiveness in various assays (Choudhary et al., 2008).
7. Enzymatic Derivatization
Enzymatic methods have been used for the derivatization of 3-(3,4-Dihydroxyphenyl)prop-2-enoic acid, indicating its versatility in chemical modifications (Mikolasch et al., 2002).
8. Supramolecular Chemistry
The compound has been utilized in the synthesis of supramolecular dendrimers, demonstrating its utility in the field of supramolecular chemistry (Percec et al., 2006).
9. Anti-inflammatory Properties
Investigations into its anti-inflammatory effects have been conducted, adding to its potential therapeutic applications (Ren et al., 2021).
10. Optical and Electronic Properties
Studies on the optical and electronic properties of derivatives of 3-(3,4-Dihydroxyphenyl)prop-2-enoic acid have been conducted, revealing its potential in the development of new materials and technologies (Sulpizio et al., 2016).
11. Novel Compound Synthesis
Research has been focused on the synthesis of new compounds derived from 3-(3,4-Dihydroxyphenyl)prop-2-enoic acid, expanding its application in various fields of chemistry (Hanzawa et al., 2012).
Mechanism of Action
Caffeic acid, CAPE, and the synthetic this compound phenethyl amide (CAPA) exhibit vasorelaxant activity by acting on the endothelial and vascular smooth muscle cells. Vasorelaxant mechanisms include the increased endothelial NO secretion, modulation of calcium and potassium channels, and modulation of adrenergic receptors .
Safety and Hazards
Properties
IUPAC Name |
3-(3,4-dihydroxyphenyl)prop-2-enoic acid | |
---|---|---|
Details | Computed by Lexichem TK 2.7.0 (PubChem release 2021.05.07) | |
Source | PubChem | |
URL | https://pubchem.ncbi.nlm.nih.gov | |
Description | Data deposited in or computed by PubChem | |
InChI |
InChI=1S/C9H8O4/c10-7-3-1-6(5-8(7)11)2-4-9(12)13/h1-5,10-11H,(H,12,13) | |
Details | Computed by InChI 1.0.6 (PubChem release 2021.05.07) | |
Source | PubChem | |
URL | https://pubchem.ncbi.nlm.nih.gov | |
Description | Data deposited in or computed by PubChem | |
InChI Key |
QAIPRVGONGVQAS-UHFFFAOYSA-N | |
Details | Computed by InChI 1.0.6 (PubChem release 2021.05.07) | |
Source | PubChem | |
URL | https://pubchem.ncbi.nlm.nih.gov | |
Description | Data deposited in or computed by PubChem | |
Canonical SMILES |
C1=CC(=C(C=C1C=CC(=O)O)O)O | |
Details | Computed by OEChem 2.3.0 (PubChem release 2021.05.07) | |
Source | PubChem | |
URL | https://pubchem.ncbi.nlm.nih.gov | |
Description | Data deposited in or computed by PubChem | |
Molecular Formula |
C9H8O4 | |
Details | Computed by PubChem 2.1 (PubChem release 2021.05.07) | |
Source | PubChem | |
URL | https://pubchem.ncbi.nlm.nih.gov | |
Description | Data deposited in or computed by PubChem | |
Related CAS |
71693-97-5 | |
Details | Compound: Caffeic acid polymer | |
Record name | Caffeic acid polymer | |
Source | CAS Common Chemistry | |
URL | https://commonchemistry.cas.org/detail?cas_rn=71693-97-5 | |
Description | CAS Common Chemistry is an open community resource for accessing chemical information. Nearly 500,000 chemical substances from CAS REGISTRY cover areas of community interest, including common and frequently regulated chemicals, and those relevant to high school and undergraduate chemistry classes. This chemical information, curated by our expert scientists, is provided in alignment with our mission as a division of the American Chemical Society. | |
Explanation | The data from CAS Common Chemistry is provided under a CC-BY-NC 4.0 license, unless otherwise stated. | |
DSSTOX Substance ID |
DTXSID5020231 | |
Record name | 3,4-Dihydroxycinnamic acid | |
Source | EPA DSSTox | |
URL | https://comptox.epa.gov/dashboard/DTXSID5020231 | |
Description | DSSTox provides a high quality public chemistry resource for supporting improved predictive toxicology. | |
Molecular Weight |
180.16 g/mol | |
Details | Computed by PubChem 2.1 (PubChem release 2021.05.07) | |
Source | PubChem | |
URL | https://pubchem.ncbi.nlm.nih.gov | |
Description | Data deposited in or computed by PubChem | |
CAS No. |
331-39-5 | |
Record name | Caffeic acid | |
Source | CAS Common Chemistry | |
URL | https://commonchemistry.cas.org/detail?cas_rn=331-39-5 | |
Description | CAS Common Chemistry is an open community resource for accessing chemical information. Nearly 500,000 chemical substances from CAS REGISTRY cover areas of community interest, including common and frequently regulated chemicals, and those relevant to high school and undergraduate chemistry classes. This chemical information, curated by our expert scientists, is provided in alignment with our mission as a division of the American Chemical Society. | |
Explanation | The data from CAS Common Chemistry is provided under a CC-BY-NC 4.0 license, unless otherwise stated. | |
Record name | 3,4-Dihydroxycinnamic acid | |
Source | EPA DSSTox | |
URL | https://comptox.epa.gov/dashboard/DTXSID5020231 | |
Description | DSSTox provides a high quality public chemistry resource for supporting improved predictive toxicology. | |
Synthesis routes and methods I
Procedure details
Synthesis routes and methods II
Procedure details
Synthesis routes and methods III
Procedure details
Retrosynthesis Analysis
AI-Powered Synthesis Planning: Our tool employs the Template_relevance Pistachio, Template_relevance Bkms_metabolic, Template_relevance Pistachio_ringbreaker, Template_relevance Reaxys, Template_relevance Reaxys_biocatalysis model, leveraging a vast database of chemical reactions to predict feasible synthetic routes.
One-Step Synthesis Focus: Specifically designed for one-step synthesis, it provides concise and direct routes for your target compounds, streamlining the synthesis process.
Accurate Predictions: Utilizing the extensive PISTACHIO, BKMS_METABOLIC, PISTACHIO_RINGBREAKER, REAXYS, REAXYS_BIOCATALYSIS database, our tool offers high-accuracy predictions, reflecting the latest in chemical research and data.
Strategy Settings
Precursor scoring | Relevance Heuristic |
---|---|
Min. plausibility | 0.01 |
Model | Template_relevance |
Template Set | Pistachio/Bkms_metabolic/Pistachio_ringbreaker/Reaxys/Reaxys_biocatalysis |
Top-N result to add to graph | 6 |
Feasible Synthetic Routes
Disclaimer and Information on In-Vitro Research Products
Please be aware that all articles and product information presented on BenchChem are intended solely for informational purposes. The products available for purchase on BenchChem are specifically designed for in-vitro studies, which are conducted outside of living organisms. In-vitro studies, derived from the Latin term "in glass," involve experiments performed in controlled laboratory settings using cells or tissues. It is important to note that these products are not categorized as medicines or drugs, and they have not received approval from the FDA for the prevention, treatment, or cure of any medical condition, ailment, or disease. We must emphasize that any form of bodily introduction of these products into humans or animals is strictly prohibited by law. It is essential to adhere to these guidelines to ensure compliance with legal and ethical standards in research and experimentation.