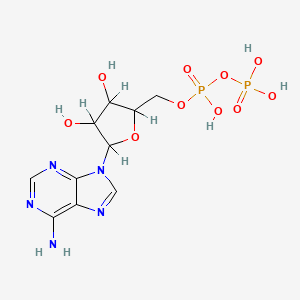
Adenosine 5'-diphosphate
Overview
Description
It consists of three key structural components: a sugar backbone attached to adenine and two phosphate groups bonded to the 5’ carbon atom of ribose . Adenosine 5’-diphosphate can be interconverted to adenosine triphosphate and adenosine monophosphate, playing a crucial role in cellular energy transfer .
Preparation Methods
Synthetic Routes and Reaction Conditions: Adenosine 5’-diphosphate can be synthesized through enzymatic and chemical methods. One common enzymatic method involves the use of ATP sulfurylase, APS kinase, and pyrophosphatase to catalyze the conversion of adenosine triphosphate to adenosine 5’-diphosphate . The reaction conditions typically include a Tris-HCl buffer (pH 8.0), magnesium chloride, and sodium sulfate, incubated at 30°C for several hours .
Industrial Production Methods: Industrial production of adenosine 5’-diphosphate often employs large-scale enzymatic synthesis due to its efficiency and specificity. The process involves the use of immobilized enzymes to catalyze the conversion of adenosine triphosphate to adenosine 5’-diphosphate under controlled conditions .
Chemical Reactions Analysis
Types of Reactions: Adenosine 5’-diphosphate undergoes various chemical reactions, including hydrolysis, phosphorylation, and dephosphorylation.
Common Reagents and Conditions:
Hydrolysis: Catalyzed by ATPases, adenosine 5’-diphosphate is hydrolyzed to adenosine monophosphate and inorganic phosphate.
Phosphorylation: Adenosine 5’-diphosphate can be phosphorylated to adenosine triphosphate using ATP synthases in the presence of inorganic phosphate and energy sources.
Major Products:
Hydrolysis: Adenosine monophosphate and inorganic phosphate.
Phosphorylation: Adenosine triphosphate.
Scientific Research Applications
Adenosine 5’-diphosphate has a wide range of applications in scientific research:
Mechanism of Action
Adenosine 5’-diphosphate exerts its effects by binding to specific receptors on the surface of cells, particularly platelets. This binding activates intracellular signaling pathways that lead to platelet activation, shape change, and recruitment of additional platelets to form a stable clot . The compound also plays a role in energy storage and nucleic acid metabolism by being converted into adenosine triphosphate by ATP synthases .
Comparison with Similar Compounds
Adenosine triphosphate (ATP): Contains three phosphate groups and is a higher energy molecule compared to adenosine 5’-diphosphate.
Adenosine monophosphate (AMP): Contains one phosphate group and is a lower energy molecule compared to adenosine 5’-diphosphate.
Uniqueness: Adenosine 5’-diphosphate is unique in its role as an intermediary in the conversion between adenosine triphosphate and adenosine monophosphate, making it essential for cellular energy transfer and metabolic regulation .
Properties
IUPAC Name |
[5-(6-aminopurin-9-yl)-3,4-dihydroxyoxolan-2-yl]methyl phosphono hydrogen phosphate | |
---|---|---|
Details | Computed by Lexichem TK 2.7.0 (PubChem release 2021.05.07) | |
Source | PubChem | |
URL | https://pubchem.ncbi.nlm.nih.gov | |
Description | Data deposited in or computed by PubChem | |
InChI |
InChI=1S/C10H15N5O10P2/c11-8-5-9(13-2-12-8)15(3-14-5)10-7(17)6(16)4(24-10)1-23-27(21,22)25-26(18,19)20/h2-4,6-7,10,16-17H,1H2,(H,21,22)(H2,11,12,13)(H2,18,19,20) | |
Details | Computed by InChI 1.0.6 (PubChem release 2021.05.07) | |
Source | PubChem | |
URL | https://pubchem.ncbi.nlm.nih.gov | |
Description | Data deposited in or computed by PubChem | |
InChI Key |
XTWYTFMLZFPYCI-UHFFFAOYSA-N | |
Details | Computed by InChI 1.0.6 (PubChem release 2021.05.07) | |
Source | PubChem | |
URL | https://pubchem.ncbi.nlm.nih.gov | |
Description | Data deposited in or computed by PubChem | |
Canonical SMILES |
C1=NC(=C2C(=N1)N(C=N2)C3C(C(C(O3)COP(=O)(O)OP(=O)(O)O)O)O)N | |
Details | Computed by OEChem 2.3.0 (PubChem release 2021.05.07) | |
Source | PubChem | |
URL | https://pubchem.ncbi.nlm.nih.gov | |
Description | Data deposited in or computed by PubChem | |
Molecular Formula |
C10H15N5O10P2 | |
Details | Computed by PubChem 2.1 (PubChem release 2021.05.07) | |
Source | PubChem | |
URL | https://pubchem.ncbi.nlm.nih.gov | |
Description | Data deposited in or computed by PubChem | |
DSSTOX Substance ID |
DTXSID30862572 | |
Record name | 9-{5-O-[Hydroxy(phosphonooxy)phosphoryl]pentofuranosyl}-9H-purin-6-amine | |
Source | EPA DSSTox | |
URL | https://comptox.epa.gov/dashboard/DTXSID30862572 | |
Description | DSSTox provides a high quality public chemistry resource for supporting improved predictive toxicology. | |
Molecular Weight |
427.20 g/mol | |
Details | Computed by PubChem 2.1 (PubChem release 2021.05.07) | |
Source | PubChem | |
URL | https://pubchem.ncbi.nlm.nih.gov | |
Description | Data deposited in or computed by PubChem | |
CAS No. |
2092-65-1 | |
Record name | Adenosine trisodium 5'-diphosphate trihydrate | |
Source | ChemIDplus | |
URL | https://pubchem.ncbi.nlm.nih.gov/substance/?source=chemidplus&sourceid=0002092651 | |
Description | ChemIDplus is a free, web search system that provides access to the structure and nomenclature authority files used for the identification of chemical substances cited in National Library of Medicine (NLM) databases, including the TOXNET system. | |
Record name | NSC124141 | |
Source | DTP/NCI | |
URL | https://dtp.cancer.gov/dtpstandard/servlet/dwindex?searchtype=NSC&outputformat=html&searchlist=124141 | |
Description | The NCI Development Therapeutics Program (DTP) provides services and resources to the academic and private-sector research communities worldwide to facilitate the discovery and development of new cancer therapeutic agents. | |
Explanation | Unless otherwise indicated, all text within NCI products is free of copyright and may be reused without our permission. Credit the National Cancer Institute as the source. | |
Record name | Adenosine trisodium 5'-diphosphate trihydrate | |
Source | European Chemicals Agency (ECHA) | |
URL | https://echa.europa.eu/substance-information/-/substanceinfo/100.016.590 | |
Description | The European Chemicals Agency (ECHA) is an agency of the European Union which is the driving force among regulatory authorities in implementing the EU's groundbreaking chemicals legislation for the benefit of human health and the environment as well as for innovation and competitiveness. | |
Explanation | Use of the information, documents and data from the ECHA website is subject to the terms and conditions of this Legal Notice, and subject to other binding limitations provided for under applicable law, the information, documents and data made available on the ECHA website may be reproduced, distributed and/or used, totally or in part, for non-commercial purposes provided that ECHA is acknowledged as the source: "Source: European Chemicals Agency, http://echa.europa.eu/". Such acknowledgement must be included in each copy of the material. ECHA permits and encourages organisations and individuals to create links to the ECHA website under the following cumulative conditions: Links can only be made to webpages that provide a link to the Legal Notice page. | |
Retrosynthesis Analysis
AI-Powered Synthesis Planning: Our tool employs the Template_relevance Pistachio, Template_relevance Bkms_metabolic, Template_relevance Pistachio_ringbreaker, Template_relevance Reaxys, Template_relevance Reaxys_biocatalysis model, leveraging a vast database of chemical reactions to predict feasible synthetic routes.
One-Step Synthesis Focus: Specifically designed for one-step synthesis, it provides concise and direct routes for your target compounds, streamlining the synthesis process.
Accurate Predictions: Utilizing the extensive PISTACHIO, BKMS_METABOLIC, PISTACHIO_RINGBREAKER, REAXYS, REAXYS_BIOCATALYSIS database, our tool offers high-accuracy predictions, reflecting the latest in chemical research and data.
Strategy Settings
Precursor scoring | Relevance Heuristic |
---|---|
Min. plausibility | 0.01 |
Model | Template_relevance |
Template Set | Pistachio/Bkms_metabolic/Pistachio_ringbreaker/Reaxys/Reaxys_biocatalysis |
Top-N result to add to graph | 6 |
Feasible Synthetic Routes
Disclaimer and Information on In-Vitro Research Products
Please be aware that all articles and product information presented on BenchChem are intended solely for informational purposes. The products available for purchase on BenchChem are specifically designed for in-vitro studies, which are conducted outside of living organisms. In-vitro studies, derived from the Latin term "in glass," involve experiments performed in controlled laboratory settings using cells or tissues. It is important to note that these products are not categorized as medicines or drugs, and they have not received approval from the FDA for the prevention, treatment, or cure of any medical condition, ailment, or disease. We must emphasize that any form of bodily introduction of these products into humans or animals is strictly prohibited by law. It is essential to adhere to these guidelines to ensure compliance with legal and ethical standards in research and experimentation.