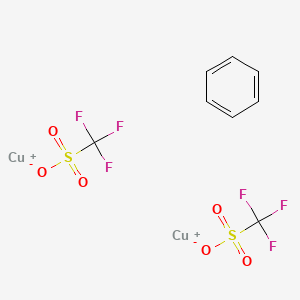
Copper(I) trifluoromethanesulfonate benzene complex
- Click on QUICK INQUIRY to receive a quote from our team of experts.
- With the quality product at a COMPETITIVE price, you can focus more on your research.
Overview
Description
Preparation Methods
Synthetic Routes and Reaction Conditions
Copper(I) trifluoromethanesulfonate benzene complex can be synthesized by reacting copper(I) oxide or copper(I) chloride with trifluoromethanesulfonic acid in the presence of benzene . The reaction typically occurs under an inert atmosphere to prevent oxidation of the copper(I) ion . The product is then purified by recrystallization from an appropriate solvent .
Industrial Production Methods
While specific industrial production methods for this compound are not widely documented, the general approach involves large-scale synthesis using similar reaction conditions as in laboratory settings. The process may include additional steps for purification and quality control to ensure the compound meets industrial standards .
Chemical Reactions Analysis
Types of Reactions
Copper(I) trifluoromethanesulfonate benzene complex is known to undergo various types of reactions, including:
Oxidation: The copper(I) ion can be oxidized to copper(II) under certain conditions.
Substitution: The benzene ligand can be substituted with other aromatic compounds.
Coordination: The copper(I) ion can coordinate with different ligands, forming new complexes.
Common Reagents and Conditions
Common reagents used in reactions with this compound include alkyl benzenes, cyclic alkenes, and N-benzoyloxysulfonamides . Reactions are typically carried out under inert atmospheres and may require specific solvents and temperatures to achieve desired outcomes .
Major Products
Major products formed from reactions involving this compound include enol-esters, 2,5-disubstituted pyrrolidine derivatives, and β-alkylcarbonyls .
Scientific Research Applications
Copper(I) trifluoromethanesulfonate benzene complex has a wide range of applications in scientific research:
Mechanism of Action
The mechanism by which copper(I) trifluoromethanesulfonate benzene complex exerts its effects involves the coordination of the copper(I) ion with various ligands, facilitating catalytic reactions . The copper(I) ion acts as a Lewis acid, activating substrates and enabling transformations that would otherwise be challenging . Molecular targets include alkenes, alkynes, and other unsaturated compounds .
Comparison with Similar Compounds
Similar Compounds
- Copper(I) trifluoromethanesulfonate toluene complex
- Copper(II) trifluoromethanesulfonate
- Tetrakis(acetonitrile)copper(I) triflate
- Tetrakis(acetonitrile)copper(I) hexafluorophosphate
Uniqueness
Copper(I) trifluoromethanesulfonate benzene complex is unique due to its specific coordination with benzene, which imparts distinct catalytic properties and reactivity patterns . Its ability to facilitate enantioselective reactions with high yield and selectivity sets it apart from other copper(I) complexes .
Properties
IUPAC Name |
benzene;copper(1+);trifluoromethanesulfonate |
Source
|
---|---|---|
Details | Computed by Lexichem TK 2.7.0 (PubChem release 2021.05.07) | |
Source | PubChem | |
URL | https://pubchem.ncbi.nlm.nih.gov | |
Description | Data deposited in or computed by PubChem | |
InChI |
InChI=1S/C6H6.2CHF3O3S.2Cu/c1-2-4-6-5-3-1;2*2-1(3,4)8(5,6)7;;/h1-6H;2*(H,5,6,7);;/q;;;2*+1/p-2 |
Source
|
Details | Computed by InChI 1.0.6 (PubChem release 2021.05.07) | |
Source | PubChem | |
URL | https://pubchem.ncbi.nlm.nih.gov | |
Description | Data deposited in or computed by PubChem | |
InChI Key |
GNXZWVVAAMVOJY-UHFFFAOYSA-L |
Source
|
Details | Computed by InChI 1.0.6 (PubChem release 2021.05.07) | |
Source | PubChem | |
URL | https://pubchem.ncbi.nlm.nih.gov | |
Description | Data deposited in or computed by PubChem | |
Canonical SMILES |
C1=CC=CC=C1.C(F)(F)(F)S(=O)(=O)[O-].C(F)(F)(F)S(=O)(=O)[O-].[Cu+].[Cu+] |
Source
|
Details | Computed by OEChem 2.3.0 (PubChem release 2021.05.07) | |
Source | PubChem | |
URL | https://pubchem.ncbi.nlm.nih.gov | |
Description | Data deposited in or computed by PubChem | |
Molecular Formula |
C8H6Cu2F6O6S2 |
Source
|
Details | Computed by PubChem 2.1 (PubChem release 2021.05.07) | |
Source | PubChem | |
URL | https://pubchem.ncbi.nlm.nih.gov | |
Description | Data deposited in or computed by PubChem | |
Molecular Weight |
503.3 g/mol |
Source
|
Details | Computed by PubChem 2.1 (PubChem release 2021.05.07) | |
Source | PubChem | |
URL | https://pubchem.ncbi.nlm.nih.gov | |
Description | Data deposited in or computed by PubChem | |
Disclaimer and Information on In-Vitro Research Products
Please be aware that all articles and product information presented on BenchChem are intended solely for informational purposes. The products available for purchase on BenchChem are specifically designed for in-vitro studies, which are conducted outside of living organisms. In-vitro studies, derived from the Latin term "in glass," involve experiments performed in controlled laboratory settings using cells or tissues. It is important to note that these products are not categorized as medicines or drugs, and they have not received approval from the FDA for the prevention, treatment, or cure of any medical condition, ailment, or disease. We must emphasize that any form of bodily introduction of these products into humans or animals is strictly prohibited by law. It is essential to adhere to these guidelines to ensure compliance with legal and ethical standards in research and experimentation.