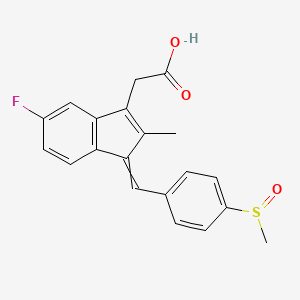
Sulindac sulfoxide
Overview
Description
Sulindac is a non-steroidal, anti-inflammatory indene derivative . It’s a nonsteroidal anti-inflammatory drug (NSAID) of the arylalkanoic acid class . It’s used to treat pain or inflammation caused by arthritis, ankylosing spondylitis, tendinitis, bursitis, or gout . It’s not a salicylate, pyrazolone or propionic acid derivative . Its empirical formula is C20H17FO3S, with a molecular weight of 356.42 .
Molecular Structure Analysis
Sulindac is a yellow crystalline compound, a weak organic acid practically insoluble in water below pH 4.5, but very soluble as the sodium salt or in buffers of pH 6 or higher . The structural formulas of sulindac and its metabolites are available .Chemical Reactions Analysis
Sulindac undergoes two major biotransformations - reversible reduction to the sulfide metabolite, and irreversible oxidation to the sulfone metabolite . The biological activity resides with the sulfide metabolite . The bioavailability of sulindac is higher for the capsule compared with the tablet formulation .Physical And Chemical Properties Analysis
Sulindac is a yellow crystalline compound . It’s a weak organic acid practically insoluble in water below pH 4.5, but very soluble as the sodium salt or in buffers of pH 6 or higher . More detailed physical and chemical properties were not found in the search results.Scientific Research Applications
Cancer Chemoprevention : Sulindac sulfoxide metabolizes into sulindac sulfone, which exhibits chemopreventive activity against mammary carcinogenesis. It was found to reduce cancer incidence and prolong cancer latency, suggesting its potential as a tissue-specific chemopreventive agent (Thompson et al., 1997).
Bioactivation and Tissue Distribution : Studies reveal that sulindac undergoes rapid metabolic interchange between its sulfoxide and sulfide forms in plasma and tissues. Kidney and liver are major sites of bioactivation, suggesting the importance of these organs in sulindac metabolism (Duggan et al., 1980).
Scavenging of Reactive Species : The metabolism of sulindac enhances its scavenging activity against reactive oxygen and nitrogen species, contributing to its anti-inflammatory and anticarcinogenic efficacy (Fernandes et al., 2003).
Angiogenesis Inhibition : Sulindac sulfoxide and its sulfone metabolite inhibit angiogenesis, which might contribute to their antineoplastic properties. This effect was observed in human lung tumor models in mice (Skopińska-Różewska et al., 1998).
Reduction to Active Metabolites : Sulindac is a pro-drug reduced to sulindac sulfide to be active as a COX inhibitor. The reduction process was studied in detail, highlighting the role of certain enzymes in this metabolic pathway (Etienne et al., 2003).
Metabolism and Biological Activity : The metabolism of sulindac and its epimers were studied for their effects on normal and lung cancer cells exposed to oxidative stress, providing insights into their potential therapeutic applications (Brunell et al., 2011).
Mechanism of Action
Safety and Hazards
Sulindac may cause an increased risk of serious cardiovascular thrombotic events, including myocardial infarction and stroke, which can be fatal . It may also cause an increased risk of serious gastrointestinal adverse events including bleeding, ulceration, and perforation of the stomach or intestines, which can be fatal . Elderly patients are at greater risk for serious gastrointestinal events . It’s recommended to handle Sulindac with personal protective equipment, avoid contact with skin, eyes and clothing, use only in well-ventilated areas, do not breathe vapors/dust, do not ingest, and keep away from heat and sources of ignition .
Future Directions
Sulindac has shown consistent chemopreventive potential in preclinical studies as well as in a limited number of clinical trials reported to date . It remains a promising candidate chemoprevention agent with potentially beneficial effects in multiple target organs . Sulindac capsules, which can be readily prepared with matching placebos, are supported for use in future blinded chemoprevention trials . There’s also ongoing research into the use of Sulindac in the treatment of triple-negative breast cancer .
properties
IUPAC Name |
2-[6-fluoro-2-methyl-3-[(4-methylsulfinylphenyl)methylidene]inden-1-yl]acetic acid | |
---|---|---|
Details | Computed by Lexichem TK 2.7.0 (PubChem release 2021.05.07) | |
Source | PubChem | |
URL | https://pubchem.ncbi.nlm.nih.gov | |
Description | Data deposited in or computed by PubChem | |
InChI |
InChI=1S/C20H17FO3S/c1-12-17(9-13-3-6-15(7-4-13)25(2)24)16-8-5-14(21)10-19(16)18(12)11-20(22)23/h3-10H,11H2,1-2H3,(H,22,23) | |
Details | Computed by InChI 1.0.6 (PubChem release 2021.05.07) | |
Source | PubChem | |
URL | https://pubchem.ncbi.nlm.nih.gov | |
Description | Data deposited in or computed by PubChem | |
InChI Key |
MLKXDPUZXIRXEP-UHFFFAOYSA-N | |
Details | Computed by InChI 1.0.6 (PubChem release 2021.05.07) | |
Source | PubChem | |
URL | https://pubchem.ncbi.nlm.nih.gov | |
Description | Data deposited in or computed by PubChem | |
Canonical SMILES |
CC1=C(C2=C(C1=CC3=CC=C(C=C3)S(=O)C)C=CC(=C2)F)CC(=O)O | |
Details | Computed by OEChem 2.3.0 (PubChem release 2021.05.07) | |
Source | PubChem | |
URL | https://pubchem.ncbi.nlm.nih.gov | |
Description | Data deposited in or computed by PubChem | |
Molecular Formula |
C20H17FO3S | |
Details | Computed by PubChem 2.1 (PubChem release 2021.05.07) | |
Source | PubChem | |
URL | https://pubchem.ncbi.nlm.nih.gov | |
Description | Data deposited in or computed by PubChem | |
Molecular Weight |
356.4 g/mol | |
Details | Computed by PubChem 2.1 (PubChem release 2021.05.07) | |
Source | PubChem | |
URL | https://pubchem.ncbi.nlm.nih.gov | |
Description | Data deposited in or computed by PubChem | |
Product Name |
2-[6-Fluoro-2-methyl-3-[(4-methylsulfinylphenyl)methylidene]-1-indenyl]acetic acid | |
CAS RN |
32004-68-5, 9000-14-0, 38194-50-2 | |
Record name | 5-Fluoro-2-methyl-1-[[4-(methylsulfinyl)phenyl]methylene]-1H-indene-3-acetic acid | |
Source | CAS Common Chemistry | |
URL | https://commonchemistry.cas.org/detail?cas_rn=32004-68-5 | |
Description | CAS Common Chemistry is an open community resource for accessing chemical information. Nearly 500,000 chemical substances from CAS REGISTRY cover areas of community interest, including common and frequently regulated chemicals, and those relevant to high school and undergraduate chemistry classes. This chemical information, curated by our expert scientists, is provided in alignment with our mission as a division of the American Chemical Society. | |
Explanation | The data from CAS Common Chemistry is provided under a CC-BY-NC 4.0 license, unless otherwise stated. | |
Record name | Copals | |
Source | EPA Chemicals under the TSCA | |
URL | https://www.epa.gov/chemicals-under-tsca | |
Description | EPA Chemicals under the Toxic Substances Control Act (TSCA) collection contains information on chemicals and their regulations under TSCA, including non-confidential content from the TSCA Chemical Substance Inventory and Chemical Data Reporting. | |
Record name | 5-fluoro-2-methyl-1-[[4-(methylsulphinyl)phenyl]methylene]-1H-indene-3-acetic acid | |
Source | European Chemicals Agency (ECHA) | |
URL | https://echa.europa.eu/substance-information/-/substanceinfo/100.046.252 | |
Description | The European Chemicals Agency (ECHA) is an agency of the European Union which is the driving force among regulatory authorities in implementing the EU's groundbreaking chemicals legislation for the benefit of human health and the environment as well as for innovation and competitiveness. | |
Explanation | Use of the information, documents and data from the ECHA website is subject to the terms and conditions of this Legal Notice, and subject to other binding limitations provided for under applicable law, the information, documents and data made available on the ECHA website may be reproduced, distributed and/or used, totally or in part, for non-commercial purposes provided that ECHA is acknowledged as the source: "Source: European Chemicals Agency, http://echa.europa.eu/". Such acknowledgement must be included in each copy of the material. ECHA permits and encourages organisations and individuals to create links to the ECHA website under the following cumulative conditions: Links can only be made to webpages that provide a link to the Legal Notice page. | |
Record name | Copals | |
Source | European Chemicals Agency (ECHA) | |
URL | https://echa.europa.eu/substance-information/-/substanceinfo/100.029.559 | |
Description | The European Chemicals Agency (ECHA) is an agency of the European Union which is the driving force among regulatory authorities in implementing the EU's groundbreaking chemicals legislation for the benefit of human health and the environment as well as for innovation and competitiveness. | |
Explanation | Use of the information, documents and data from the ECHA website is subject to the terms and conditions of this Legal Notice, and subject to other binding limitations provided for under applicable law, the information, documents and data made available on the ECHA website may be reproduced, distributed and/or used, totally or in part, for non-commercial purposes provided that ECHA is acknowledged as the source: "Source: European Chemicals Agency, http://echa.europa.eu/". Such acknowledgement must be included in each copy of the material. ECHA permits and encourages organisations and individuals to create links to the ECHA website under the following cumulative conditions: Links can only be made to webpages that provide a link to the Legal Notice page. | |
Record name | Sulindac | |
Source | European Chemicals Agency (ECHA) | |
URL | https://echa.europa.eu/substance-information/-/substanceinfo/100.048.909 | |
Description | The European Chemicals Agency (ECHA) is an agency of the European Union which is the driving force among regulatory authorities in implementing the EU's groundbreaking chemicals legislation for the benefit of human health and the environment as well as for innovation and competitiveness. | |
Explanation | Use of the information, documents and data from the ECHA website is subject to the terms and conditions of this Legal Notice, and subject to other binding limitations provided for under applicable law, the information, documents and data made available on the ECHA website may be reproduced, distributed and/or used, totally or in part, for non-commercial purposes provided that ECHA is acknowledged as the source: "Source: European Chemicals Agency, http://echa.europa.eu/". Such acknowledgement must be included in each copy of the material. ECHA permits and encourages organisations and individuals to create links to the ECHA website under the following cumulative conditions: Links can only be made to webpages that provide a link to the Legal Notice page. | |
Retrosynthesis Analysis
AI-Powered Synthesis Planning: Our tool employs the Template_relevance Pistachio, Template_relevance Bkms_metabolic, Template_relevance Pistachio_ringbreaker, Template_relevance Reaxys, Template_relevance Reaxys_biocatalysis model, leveraging a vast database of chemical reactions to predict feasible synthetic routes.
One-Step Synthesis Focus: Specifically designed for one-step synthesis, it provides concise and direct routes for your target compounds, streamlining the synthesis process.
Accurate Predictions: Utilizing the extensive PISTACHIO, BKMS_METABOLIC, PISTACHIO_RINGBREAKER, REAXYS, REAXYS_BIOCATALYSIS database, our tool offers high-accuracy predictions, reflecting the latest in chemical research and data.
Strategy Settings
Precursor scoring | Relevance Heuristic |
---|---|
Min. plausibility | 0.01 |
Model | Template_relevance |
Template Set | Pistachio/Bkms_metabolic/Pistachio_ringbreaker/Reaxys/Reaxys_biocatalysis |
Top-N result to add to graph | 6 |
Feasible Synthetic Routes
Disclaimer and Information on In-Vitro Research Products
Please be aware that all articles and product information presented on BenchChem are intended solely for informational purposes. The products available for purchase on BenchChem are specifically designed for in-vitro studies, which are conducted outside of living organisms. In-vitro studies, derived from the Latin term "in glass," involve experiments performed in controlled laboratory settings using cells or tissues. It is important to note that these products are not categorized as medicines or drugs, and they have not received approval from the FDA for the prevention, treatment, or cure of any medical condition, ailment, or disease. We must emphasize that any form of bodily introduction of these products into humans or animals is strictly prohibited by law. It is essential to adhere to these guidelines to ensure compliance with legal and ethical standards in research and experimentation.