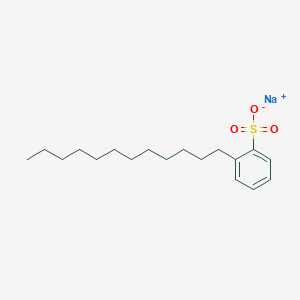
sodium;2-dodecylbenzenesulfonate
Overview
Description
Cyclodextrin . Cyclodextrins are a family of cyclic oligosaccharides composed of glucose monomers arranged in a ring. They are produced from starch by enzymatic conversion and are known for their ability to form inclusion complexes with various molecules, enhancing the solubility and stability of these molecules.
Preparation Methods
Synthetic Routes and Reaction Conditions
Cyclodextrins are synthesized from starch through the action of cyclodextrin glycosyltransferase (CGTase) enzymes. The process involves the enzymatic conversion of starch into cyclodextrins under controlled conditions. The reaction typically occurs in an aqueous medium at a pH of around 5-6 and a temperature of 50-60°C. The reaction mixture is then purified to isolate the desired cyclodextrin.
Industrial Production Methods
Industrial production of cyclodextrins involves the use of large-scale bioreactors where starch is enzymatically converted into cyclodextrins. The process includes steps such as liquefaction of starch, enzymatic conversion, and purification. The purified cyclodextrins are then dried and packaged for various applications.
Chemical Reactions Analysis
Types of Reactions
Cyclodextrins undergo various chemical reactions, including:
Oxidation: Cyclodextrins can be oxidized to form derivatives with different functional groups.
Reduction: Reduction reactions can modify the hydroxyl groups on the cyclodextrin ring.
Substitution: Substitution reactions can introduce different substituents onto the cyclodextrin ring, enhancing its properties.
Common Reagents and Conditions
Oxidation: Common oxidizing agents include sodium periodate and hydrogen peroxide.
Reduction: Reducing agents such as sodium borohydride are used.
Substitution: Reagents like alkyl halides and acyl chlorides are used for substitution reactions.
Major Products Formed
The major products formed from these reactions include various cyclodextrin derivatives with enhanced solubility, stability, and inclusion complex formation capabilities.
Scientific Research Applications
Cyclodextrins have a wide range of scientific research applications, including:
Chemistry: Used as molecular carriers to enhance the solubility and stability of hydrophobic compounds.
Biology: Employed in drug delivery systems to improve the bioavailability of pharmaceuticals.
Medicine: Used in formulations to enhance the delivery and efficacy of drugs.
Industry: Applied in food, cosmetics, and environmental industries for encapsulation and stabilization of active ingredients.
Mechanism of Action
Cyclodextrins exert their effects through the formation of inclusion complexes with guest molecules. The hydrophobic cavity of the cyclodextrin molecule can encapsulate hydrophobic guest molecules, while the hydrophilic exterior interacts with the aqueous environment. This inclusion complex formation enhances the solubility, stability, and bioavailability of the guest molecules.
Comparison with Similar Compounds
Similar Compounds
- Alpha-Cyclodextrin
- Beta-Cyclodextrin
- Gamma-Cyclodextrin
Uniqueness
Cyclodextrins are unique due to their ability to form inclusion complexes with a wide range of molecules. This property makes them valuable in various applications, including drug delivery, food industry, and environmental protection. Compared to other similar compounds, cyclodextrins offer a versatile and efficient means of enhancing the properties of guest molecules.
Properties
IUPAC Name |
sodium;2-dodecylbenzenesulfonate | |
---|---|---|
Source | PubChem | |
URL | https://pubchem.ncbi.nlm.nih.gov | |
Description | Data deposited in or computed by PubChem | |
InChI |
InChI=1S/C18H30O3S.Na/c1-2-3-4-5-6-7-8-9-10-11-14-17-15-12-13-16-18(17)22(19,20)21;/h12-13,15-16H,2-11,14H2,1H3,(H,19,20,21);/q;+1/p-1 | |
Source | PubChem | |
URL | https://pubchem.ncbi.nlm.nih.gov | |
Description | Data deposited in or computed by PubChem | |
InChI Key |
HFQQZARZPUDIFP-UHFFFAOYSA-M | |
Source | PubChem | |
URL | https://pubchem.ncbi.nlm.nih.gov | |
Description | Data deposited in or computed by PubChem | |
Canonical SMILES |
CCCCCCCCCCCCC1=CC=CC=C1S(=O)(=O)[O-].[Na+] | |
Source | PubChem | |
URL | https://pubchem.ncbi.nlm.nih.gov | |
Description | Data deposited in or computed by PubChem | |
Isomeric SMILES |
CCCCCCCCCCCCC1=CC=CC=C1S(=O)(=O)[O-].[Na+] | |
Source | PubChem | |
URL | https://pubchem.ncbi.nlm.nih.gov | |
Description | Data deposited in or computed by PubChem | |
Molecular Formula |
C18H29NaO3S | |
Source | PubChem | |
URL | https://pubchem.ncbi.nlm.nih.gov | |
Description | Data deposited in or computed by PubChem | |
Molecular Weight |
348.5 g/mol | |
Source | PubChem | |
URL | https://pubchem.ncbi.nlm.nih.gov | |
Description | Data deposited in or computed by PubChem | |
Synthesis routes and methods
Procedure details
Disclaimer and Information on In-Vitro Research Products
Please be aware that all articles and product information presented on BenchChem are intended solely for informational purposes. The products available for purchase on BenchChem are specifically designed for in-vitro studies, which are conducted outside of living organisms. In-vitro studies, derived from the Latin term "in glass," involve experiments performed in controlled laboratory settings using cells or tissues. It is important to note that these products are not categorized as medicines or drugs, and they have not received approval from the FDA for the prevention, treatment, or cure of any medical condition, ailment, or disease. We must emphasize that any form of bodily introduction of these products into humans or animals is strictly prohibited by law. It is essential to adhere to these guidelines to ensure compliance with legal and ethical standards in research and experimentation.