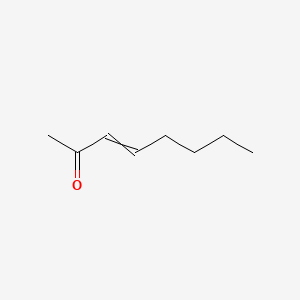
Oct-3-en-2-one
Overview
Description
Oct-3-en-2-one, also known as 3-octen-2-one, is an organic compound with the molecular formula C₈H₁₄O. It belongs to the class of enones, which are compounds containing the enone functional group, characterized by a carbon-carbon double bond conjugated with a carbonyl group. This compound is known for its distinctive odor and is used in various applications, including flavoring and fragrance industries .
Scientific Research Applications
Oct-3-en-2-one has several applications in scientific research:
Chemistry: It is used as a starting material for the synthesis of more complex organic compounds. Its reactivity makes it a valuable intermediate in organic synthesis.
Biology: The compound is studied for its potential biological activities, including antimicrobial and antifungal properties.
Medicine: Research is ongoing to explore its potential therapeutic applications, particularly in the development of new drugs.
Industry: this compound is used in the flavor and fragrance industry due to its pleasant odor. .
Preparation Methods
Synthetic Routes and Reaction Conditions: Oct-3-en-2-one can be synthesized through several methods. One common synthetic route involves the aldol condensation of hexanal with acetone, followed by dehydration to form the enone structure. The reaction typically requires a base catalyst such as sodium hydroxide or potassium hydroxide and is carried out under controlled temperature conditions to ensure the desired product is obtained.
Industrial Production Methods: In industrial settings, this compound can be produced through the catalytic dehydrogenation of octan-2-ol. This process involves the use of metal catalysts such as copper or palladium at elevated temperatures. The dehydrogenation reaction removes hydrogen atoms from octan-2-ol, resulting in the formation of this compound .
Types of Reactions:
Oxidation: this compound can undergo oxidation reactions to form various oxidation products. For example, it can be oxidized to form oct-3-en-2-ol using oxidizing agents such as potassium permanganate or chromium trioxide.
Reduction: Reduction of this compound can yield octan-2-ol. This reaction typically involves the use of reducing agents such as sodium borohydride or lithium aluminum hydride.
Substitution: The compound can participate in nucleophilic substitution reactions, where the carbonyl group can be attacked by nucleophiles, leading to the formation of substituted products.
Common Reagents and Conditions:
Oxidation: Potassium permanganate, chromium trioxide, and other strong oxidizing agents.
Reduction: Sodium borohydride, lithium aluminum hydride, and catalytic hydrogenation.
Substitution: Nucleophiles such as amines, alcohols, and thiols under acidic or basic conditions.
Major Products Formed:
Oxidation: Oct-3-en-2-ol and other oxidized derivatives.
Reduction: Octan-2-ol.
Substitution: Various substituted enone derivatives depending on the nucleophile used
Mechanism of Action
The mechanism of action of oct-3-en-2-one involves its interaction with various molecular targets. The carbonyl group in the enone structure is highly reactive and can form covalent bonds with nucleophilic sites in biological molecules. This reactivity underlies its antimicrobial and antifungal properties, as it can disrupt the function of essential enzymes and proteins in microorganisms. Additionally, the compound’s ability to undergo various chemical reactions makes it a versatile intermediate in organic synthesis .
Comparison with Similar Compounds
3-Octen-2-one (E)-: This is a stereoisomer of oct-3-en-2-one with a different spatial arrangement of atoms around the double bond.
3-Octen-2-one (Z)-: Another stereoisomer with a different configuration around the double bond.
Octan-2-one: A saturated ketone with a similar carbon chain length but lacking the double bond.
Uniqueness: this compound is unique due to its enone structure, which imparts distinct reactivity compared to its saturated counterparts. The presence of the double bond conjugated with the carbonyl group allows for a variety of chemical transformations that are not possible with saturated ketones. This makes this compound a valuable compound in both synthetic chemistry and industrial applications .
Properties
IUPAC Name |
oct-3-en-2-one | |
---|---|---|
Details | Computed by Lexichem TK 2.7.0 (PubChem release 2021.05.07) | |
Source | PubChem | |
URL | https://pubchem.ncbi.nlm.nih.gov | |
Description | Data deposited in or computed by PubChem | |
InChI |
InChI=1S/C8H14O/c1-3-4-5-6-7-8(2)9/h6-7H,3-5H2,1-2H3 | |
Details | Computed by InChI 1.0.6 (PubChem release 2021.05.07) | |
Source | PubChem | |
URL | https://pubchem.ncbi.nlm.nih.gov | |
Description | Data deposited in or computed by PubChem | |
InChI Key |
ZCFOBLITZWHNNC-UHFFFAOYSA-N | |
Details | Computed by InChI 1.0.6 (PubChem release 2021.05.07) | |
Source | PubChem | |
URL | https://pubchem.ncbi.nlm.nih.gov | |
Description | Data deposited in or computed by PubChem | |
Canonical SMILES |
CCCCC=CC(=O)C | |
Details | Computed by OEChem 2.3.0 (PubChem release 2021.05.07) | |
Source | PubChem | |
URL | https://pubchem.ncbi.nlm.nih.gov | |
Description | Data deposited in or computed by PubChem | |
Molecular Formula |
C8H14O | |
Details | Computed by PubChem 2.1 (PubChem release 2021.05.07) | |
Source | PubChem | |
URL | https://pubchem.ncbi.nlm.nih.gov | |
Description | Data deposited in or computed by PubChem | |
DSSTOX Substance ID |
DTXSID1061867 | |
Record name | 3-Octen-2-one | |
Source | EPA DSSTox | |
URL | https://comptox.epa.gov/dashboard/DTXSID1061867 | |
Description | DSSTox provides a high quality public chemistry resource for supporting improved predictive toxicology. | |
Molecular Weight |
126.20 g/mol | |
Details | Computed by PubChem 2.1 (PubChem release 2021.05.07) | |
Source | PubChem | |
URL | https://pubchem.ncbi.nlm.nih.gov | |
Description | Data deposited in or computed by PubChem | |
CAS No. |
1669-44-9 | |
Record name | 3-Octen-2-one | |
Source | CAS Common Chemistry | |
URL | https://commonchemistry.cas.org/detail?cas_rn=1669-44-9 | |
Description | CAS Common Chemistry is an open community resource for accessing chemical information. Nearly 500,000 chemical substances from CAS REGISTRY cover areas of community interest, including common and frequently regulated chemicals, and those relevant to high school and undergraduate chemistry classes. This chemical information, curated by our expert scientists, is provided in alignment with our mission as a division of the American Chemical Society. | |
Explanation | The data from CAS Common Chemistry is provided under a CC-BY-NC 4.0 license, unless otherwise stated. | |
Record name | 3-Octen-2-one | |
Source | EPA DSSTox | |
URL | https://comptox.epa.gov/dashboard/DTXSID1061867 | |
Description | DSSTox provides a high quality public chemistry resource for supporting improved predictive toxicology. | |
Record name | Oct-3-en-2-one | |
Source | European Chemicals Agency (ECHA) | |
URL | https://echa.europa.eu/substance-information/-/substanceinfo/100.015.268 | |
Description | The European Chemicals Agency (ECHA) is an agency of the European Union which is the driving force among regulatory authorities in implementing the EU's groundbreaking chemicals legislation for the benefit of human health and the environment as well as for innovation and competitiveness. | |
Explanation | Use of the information, documents and data from the ECHA website is subject to the terms and conditions of this Legal Notice, and subject to other binding limitations provided for under applicable law, the information, documents and data made available on the ECHA website may be reproduced, distributed and/or used, totally or in part, for non-commercial purposes provided that ECHA is acknowledged as the source: "Source: European Chemicals Agency, http://echa.europa.eu/". Such acknowledgement must be included in each copy of the material. ECHA permits and encourages organisations and individuals to create links to the ECHA website under the following cumulative conditions: Links can only be made to webpages that provide a link to the Legal Notice page. | |
Retrosynthesis Analysis
AI-Powered Synthesis Planning: Our tool employs the Template_relevance Pistachio, Template_relevance Bkms_metabolic, Template_relevance Pistachio_ringbreaker, Template_relevance Reaxys, Template_relevance Reaxys_biocatalysis model, leveraging a vast database of chemical reactions to predict feasible synthetic routes.
One-Step Synthesis Focus: Specifically designed for one-step synthesis, it provides concise and direct routes for your target compounds, streamlining the synthesis process.
Accurate Predictions: Utilizing the extensive PISTACHIO, BKMS_METABOLIC, PISTACHIO_RINGBREAKER, REAXYS, REAXYS_BIOCATALYSIS database, our tool offers high-accuracy predictions, reflecting the latest in chemical research and data.
Strategy Settings
Precursor scoring | Relevance Heuristic |
---|---|
Min. plausibility | 0.01 |
Model | Template_relevance |
Template Set | Pistachio/Bkms_metabolic/Pistachio_ringbreaker/Reaxys/Reaxys_biocatalysis |
Top-N result to add to graph | 6 |
Feasible Synthetic Routes
Disclaimer and Information on In-Vitro Research Products
Please be aware that all articles and product information presented on BenchChem are intended solely for informational purposes. The products available for purchase on BenchChem are specifically designed for in-vitro studies, which are conducted outside of living organisms. In-vitro studies, derived from the Latin term "in glass," involve experiments performed in controlled laboratory settings using cells or tissues. It is important to note that these products are not categorized as medicines or drugs, and they have not received approval from the FDA for the prevention, treatment, or cure of any medical condition, ailment, or disease. We must emphasize that any form of bodily introduction of these products into humans or animals is strictly prohibited by law. It is essential to adhere to these guidelines to ensure compliance with legal and ethical standards in research and experimentation.