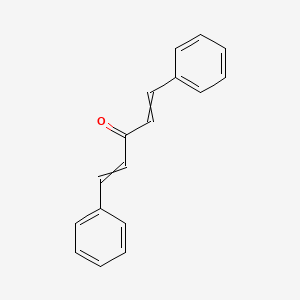
Dibenzylideneacetone
Overview
Description
Dibenzylideneacetone is an organic compound with the molecular formula C₁₇H₁₄O and a molecular weight of 234.2925 g/mol . It is also known by other names such as dibenzalacetone, bis(2-phenylvinyl) ketone, and distyryl ketone . This compound is characterized by its conjugated diene system and is often used in various chemical reactions and applications due to its unique structure.
Preparation Methods
Dibenzylideneacetone can be synthesized through the Claisen-Schmidt condensation reaction between benzaldehyde and acetone . The reaction is typically carried out in the presence of a base such as sodium hydroxide in an aqueous-alcoholic solution. The mixture is stirred vigorously at a temperature of 20-25°C, resulting in the formation of a yellow precipitate, which is then filtered and purified .
Chemical Reactions Analysis
Dibenzylideneacetone undergoes various chemical reactions, including:
Oxidation: This compound can be oxidized to form corresponding carboxylic acids or other oxidized derivatives.
Reduction: Reduction reactions can convert it into alcohols or other reduced forms.
Substitution: It can undergo substitution reactions where one or more hydrogen atoms are replaced by other atoms or groups.
Cyclization: It can participate in cyclization reactions to form cyclic compounds.
Common reagents used in these reactions include oxidizing agents like potassium permanganate, reducing agents like sodium borohydride, and various catalysts for cyclization reactions .
Scientific Research Applications
Dibenzylideneacetone has a wide range of applications in scientific research:
Chemistry: It is used as a starting material or intermediate in the synthesis of various organic compounds.
Medicine: Research has explored its potential use in drug development and therapeutic applications.
Industry: It is used in the production of polymers, resins, and other industrial chemicals.
Mechanism of Action
The mechanism of action of Dibenzylideneacetone involves its interaction with various molecular targets and pathways. Its conjugated diene system allows it to participate in electron transfer reactions, making it a useful compound in redox reactions. It can also interact with enzymes and proteins, affecting their function and activity .
Comparison with Similar Compounds
Dibenzylideneacetone can be compared with other similar compounds such as:
This compound: Another name for the same compound, highlighting its structural features.
Bis(2-phenylvinyl) ketone: Emphasizes the presence of phenyl groups attached to the vinyl moiety.
Distyryl ketone: Another synonym that reflects its chemical structure.
These compounds share similar chemical properties and reactivity due to their conjugated diene systems, but their specific applications and reactivity may vary based on their structural nuances .
Properties
IUPAC Name |
1,5-diphenylpenta-1,4-dien-3-one | |
---|---|---|
Details | Computed by Lexichem TK 2.7.0 (PubChem release 2021.05.07) | |
Source | PubChem | |
URL | https://pubchem.ncbi.nlm.nih.gov | |
Description | Data deposited in or computed by PubChem | |
InChI |
InChI=1S/C17H14O/c18-17(13-11-15-7-3-1-4-8-15)14-12-16-9-5-2-6-10-16/h1-14H | |
Details | Computed by InChI 1.0.6 (PubChem release 2021.05.07) | |
Source | PubChem | |
URL | https://pubchem.ncbi.nlm.nih.gov | |
Description | Data deposited in or computed by PubChem | |
InChI Key |
WMKGGPCROCCUDY-UHFFFAOYSA-N | |
Details | Computed by InChI 1.0.6 (PubChem release 2021.05.07) | |
Source | PubChem | |
URL | https://pubchem.ncbi.nlm.nih.gov | |
Description | Data deposited in or computed by PubChem | |
Canonical SMILES |
C1=CC=C(C=C1)C=CC(=O)C=CC2=CC=CC=C2 | |
Details | Computed by OEChem 2.3.0 (PubChem release 2021.05.07) | |
Source | PubChem | |
URL | https://pubchem.ncbi.nlm.nih.gov | |
Description | Data deposited in or computed by PubChem | |
Molecular Formula |
C17H14O | |
Details | Computed by PubChem 2.1 (PubChem release 2021.05.07) | |
Source | PubChem | |
URL | https://pubchem.ncbi.nlm.nih.gov | |
Description | Data deposited in or computed by PubChem | |
Molecular Weight |
234.29 g/mol | |
Details | Computed by PubChem 2.1 (PubChem release 2021.05.07) | |
Source | PubChem | |
URL | https://pubchem.ncbi.nlm.nih.gov | |
Description | Data deposited in or computed by PubChem | |
CAS No. |
538-58-9 | |
Record name | Dibenzylideneacetone | |
Source | CAS Common Chemistry | |
URL | https://commonchemistry.cas.org/detail?cas_rn=538-58-9 | |
Description | CAS Common Chemistry is an open community resource for accessing chemical information. Nearly 500,000 chemical substances from CAS REGISTRY cover areas of community interest, including common and frequently regulated chemicals, and those relevant to high school and undergraduate chemistry classes. This chemical information, curated by our expert scientists, is provided in alignment with our mission as a division of the American Chemical Society. | |
Explanation | The data from CAS Common Chemistry is provided under a CC-BY-NC 4.0 license, unless otherwise stated. | |
Record name | 1,4-Pentadien-3-one, 1,5-diphenyl- | |
Source | EPA Chemicals under the TSCA | |
URL | https://www.epa.gov/chemicals-under-tsca | |
Description | EPA Chemicals under the Toxic Substances Control Act (TSCA) collection contains information on chemicals and their regulations under TSCA, including non-confidential content from the TSCA Chemical Substance Inventory and Chemical Data Reporting. | |
Synthesis routes and methods I
Procedure details
Synthesis routes and methods II
Procedure details
Synthesis routes and methods III
Procedure details
Retrosynthesis Analysis
AI-Powered Synthesis Planning: Our tool employs the Template_relevance Pistachio, Template_relevance Bkms_metabolic, Template_relevance Pistachio_ringbreaker, Template_relevance Reaxys, Template_relevance Reaxys_biocatalysis model, leveraging a vast database of chemical reactions to predict feasible synthetic routes.
One-Step Synthesis Focus: Specifically designed for one-step synthesis, it provides concise and direct routes for your target compounds, streamlining the synthesis process.
Accurate Predictions: Utilizing the extensive PISTACHIO, BKMS_METABOLIC, PISTACHIO_RINGBREAKER, REAXYS, REAXYS_BIOCATALYSIS database, our tool offers high-accuracy predictions, reflecting the latest in chemical research and data.
Strategy Settings
Precursor scoring | Relevance Heuristic |
---|---|
Min. plausibility | 0.01 |
Model | Template_relevance |
Template Set | Pistachio/Bkms_metabolic/Pistachio_ringbreaker/Reaxys/Reaxys_biocatalysis |
Top-N result to add to graph | 6 |
Feasible Synthetic Routes
Disclaimer and Information on In-Vitro Research Products
Please be aware that all articles and product information presented on BenchChem are intended solely for informational purposes. The products available for purchase on BenchChem are specifically designed for in-vitro studies, which are conducted outside of living organisms. In-vitro studies, derived from the Latin term "in glass," involve experiments performed in controlled laboratory settings using cells or tissues. It is important to note that these products are not categorized as medicines or drugs, and they have not received approval from the FDA for the prevention, treatment, or cure of any medical condition, ailment, or disease. We must emphasize that any form of bodily introduction of these products into humans or animals is strictly prohibited by law. It is essential to adhere to these guidelines to ensure compliance with legal and ethical standards in research and experimentation.