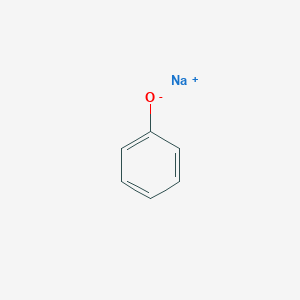
sodium;phenoxide
Overview
Description
Cyclodextrin . Cyclodextrins are a family of cyclic oligosaccharides composed of glucose monomers linked by alpha-1,4-glycosidic bonds. They are produced from starch by enzymatic conversion and are known for their ability to form inclusion complexes with various molecules, enhancing the solubility and stability of these molecules.
Preparation Methods
Synthetic Routes and Reaction Conditions
Cyclodextrins are typically synthesized through the enzymatic degradation of starch using cyclodextrin glycosyltransferase. The process involves the following steps:
Liquefaction: Starch is gelatinized and partially hydrolyzed using alpha-amylase.
Cyclization: The liquefied starch is treated with cyclodextrin glycosyltransferase, which converts the linear starch chains into cyclic cyclodextrins.
Purification: The resulting mixture is purified to isolate the desired cyclodextrin.
Industrial Production Methods
Industrial production of cyclodextrins involves large-scale enzymatic processes. The key steps include:
Starch Source: Corn, potato, or other starch sources are used.
Enzymatic Conversion: Cyclodextrin glycosyltransferase is added to the starch slurry to produce cyclodextrins.
Separation and Purification: Techniques such as crystallization, filtration, and chromatography are used to purify the cyclodextrins.
Chemical Reactions Analysis
Types of Reactions
Cyclodextrins undergo various chemical reactions, including:
Oxidation: Cyclodextrins can be oxidized to introduce functional groups such as carboxyl or aldehyde groups.
Reduction: Reduction reactions can modify the hydroxyl groups on the cyclodextrin molecules.
Substitution: Substitution reactions can introduce different functional groups onto the cyclodextrin molecule, enhancing its properties.
Common Reagents and Conditions
Oxidation: Reagents such as sodium periodate or potassium permanganate are used under mild conditions.
Reduction: Sodium borohydride or lithium aluminum hydride are common reducing agents.
Substitution: Various reagents, including alkyl halides and acyl chlorides, are used to introduce functional groups.
Major Products Formed
The major products formed from these reactions include modified cyclodextrins with enhanced solubility, stability, and functional properties.
Scientific Research Applications
Cyclodextrins have a wide range of scientific research applications:
Chemistry: Cyclodextrins are used as molecular carriers to enhance the solubility and stability of hydrophobic compounds.
Biology: They are used in drug delivery systems to improve the bioavailability of pharmaceuticals.
Medicine: Cyclodextrins are used in formulations to enhance the solubility of poorly soluble drugs.
Industry: They are used in the food industry to encapsulate flavors and fragrances, improving their stability and release properties.
Mechanism of Action
Cyclodextrins exert their effects through the formation of inclusion complexes. The hydrophobic cavity of the cyclodextrin molecule can encapsulate hydrophobic guest molecules, while the hydrophilic exterior interacts with the aqueous environment. This inclusion complex formation enhances the solubility and stability of the guest molecules, facilitating their use in various applications.
Comparison with Similar Compounds
Cyclodextrins are unique due to their ability to form inclusion complexes with a wide range of molecules. Similar compounds include:
Calixarenes: These are cyclic oligomers of phenol and formaldehyde, known for their ability to form host-guest complexes.
Cucurbiturils: These are macrocyclic molecules that can encapsulate guest molecules within their cavity.
Crown Ethers: These are cyclic compounds that can form complexes with metal ions.
Cyclodextrins are distinct from these compounds due to their biocompatibility, ease of production, and versatility in forming inclusion complexes with a wide range of guest molecules.
Properties
IUPAC Name |
sodium;phenoxide | |
---|---|---|
Source | PubChem | |
URL | https://pubchem.ncbi.nlm.nih.gov | |
Description | Data deposited in or computed by PubChem | |
InChI |
InChI=1S/C6H6O.Na/c7-6-4-2-1-3-5-6;/h1-5,7H;/q;+1/p-1 | |
Source | PubChem | |
URL | https://pubchem.ncbi.nlm.nih.gov | |
Description | Data deposited in or computed by PubChem | |
InChI Key |
NESLWCLHZZISNB-UHFFFAOYSA-M | |
Source | PubChem | |
URL | https://pubchem.ncbi.nlm.nih.gov | |
Description | Data deposited in or computed by PubChem | |
Canonical SMILES |
C1=CC=C(C=C1)[O-].[Na+] | |
Source | PubChem | |
URL | https://pubchem.ncbi.nlm.nih.gov | |
Description | Data deposited in or computed by PubChem | |
Isomeric SMILES |
C1=CC=C(C=C1)[O-].[Na+] | |
Source | PubChem | |
URL | https://pubchem.ncbi.nlm.nih.gov | |
Description | Data deposited in or computed by PubChem | |
Molecular Formula |
C6H5NaO | |
Source | PubChem | |
URL | https://pubchem.ncbi.nlm.nih.gov | |
Description | Data deposited in or computed by PubChem | |
Molecular Weight |
116.09 g/mol | |
Source | PubChem | |
URL | https://pubchem.ncbi.nlm.nih.gov | |
Description | Data deposited in or computed by PubChem | |
Synthesis routes and methods I
Procedure details
Synthesis routes and methods II
Procedure details
Synthesis routes and methods III
Procedure details
Disclaimer and Information on In-Vitro Research Products
Please be aware that all articles and product information presented on BenchChem are intended solely for informational purposes. The products available for purchase on BenchChem are specifically designed for in-vitro studies, which are conducted outside of living organisms. In-vitro studies, derived from the Latin term "in glass," involve experiments performed in controlled laboratory settings using cells or tissues. It is important to note that these products are not categorized as medicines or drugs, and they have not received approval from the FDA for the prevention, treatment, or cure of any medical condition, ailment, or disease. We must emphasize that any form of bodily introduction of these products into humans or animals is strictly prohibited by law. It is essential to adhere to these guidelines to ensure compliance with legal and ethical standards in research and experimentation.