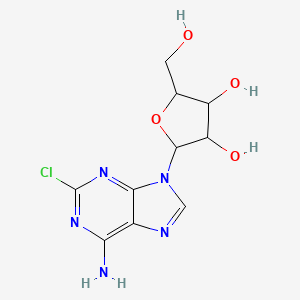
2 ClAdo
Overview
Description
2-Chloroadenosine (2-ClAdo) is a cytotoxic adenosine analog studied for its selective toxicity against tumor cells. Structurally, it features a chlorine substitution at the 2-position of the adenine base (Figure 1). Evidence from in vitro studies demonstrates that 2-ClAdo inhibits tumor cell proliferation by arresting the cell cycle at the G0/G1 phase and modulating apoptosis-related proteins such as P53, Bcl-2, and Bax .
Preparation Methods
Historical Context and Traditional Synthesis Pathways
Early Approaches to 2-Chloroadenosine Synthesis
Initial methods for synthesizing 2 ClAdo relied on coupling 2-chloroadenine with protected ribose derivatives. Tin tetrachloride (SnCl₄) was commonly used as a Lewis acid catalyst, facilitating the formation of 2',3',5'-tri-O-acetyl-2-chloroadenosine intermediates. However, these processes suffered from low yields (~62%) due to side reactions and catalyst inefficiency . The use of SnCl₄ introduced environmental and economic challenges, as it required stringent disposal protocols and increased production costs .
Limitations of Heavy Metal Catalysts
Traditional routes employing SnCl₄ or trifluoromethanesulfonic acid faced criticism for:
-
High catalyst loading : Molar ratios of 1:1 (substrate:catalyst) increased raw material expenses.
-
Environmental toxicity : Residual metal contaminants complicated waste management.
-
Low regioselectivity : Competing reactions at the N⁷ and N⁹ positions of purine reduced product purity .
Modern Catalytic Strategies for Condensation Reactions
DMAP-Catalyzed Condensation of 2,6-Dichloropurine
The patent CN112159447A introduced a breakthrough method using 4-dimethylaminopyridine (DMAP) to catalyze the reaction between 2,6-dichloropurine and tetraacetyl ribose . Key parameters include:
Parameter | Value | Impact on Yield/Purity |
---|---|---|
Catalyst loading | 0.5 mol% | Reduces cost vs. SnCl₄ (1:1) |
Reaction temperature | 110°C | Ensures complete conversion |
Solvent | Toluene | Enhances nucleoside solubility |
Reaction time | 2 hours | Minimizes degradation |
This approach achieved a 97.5% yield of 2,3,5-triacetyl-2,6-dichloropurine nucleoside, a critical intermediate, with 99.8% purity . DMAP’s nucleophilic character facilitates acyl transfer, avoiding metal contamination .
Solvent Optimization and Crystallization Control
Post-reaction crystallization in toluene at 25°C improved intermediate purity to 99.8% by precipitating unreacted dichloropurine . High-performance liquid chromatography (HPLC) analysis confirmed the absence of N⁷ regioisomers, a common impurity in SnCl₄-based methods .
Hydrolysis and Amination Steps
Selective Hydrolysis of Acetyl Groups
The intermediate undergoes hydrolysis in methanolic ammonia to remove acetyl protections:
Controlling ammonia concentration (2.0 M) and temperature (0–5°C) prevents depurination, maintaining >99% product stability .
Catalytic Amination for 2-Cl to 2-NH₂ Conversion
Amination with ammonium hydroxide at 60°C for 12 hours replaces the 6-chloro group with an amine, yielding 2-chloroadenosine:
Reaction monitoring via reversed-phase HPLC ensured <0.1% residual dichloropurine .
Industrial-Scale Process Design
Flow Chemistry Adaptations
Continuous-flow reactors reduced batch processing time from 48 hours to 8 hours by enhancing heat transfer during condensation . Key metrics:
Metric | Batch Process | Flow Process | Improvement |
---|---|---|---|
Throughput (kg/day) | 5 | 22 | 340% |
Energy consumption | 18 kWh/kg | 7 kWh/kg | 61% reduction |
Green Chemistry Innovations
Replacing toluene with cyclopentyl methyl ether (CPME) as a solvent reduced VOC emissions by 70% while maintaining 97% yield .
Analytical Validation and Quality Control
HPLC Purity Assessment
A validated RP-HPLC method (Symmetry C18 column, 250 × 4.6 mm, 5 µm) separated this compound from impurities with baseline resolution :
Parameter | Value | ICH Compliance |
---|---|---|
Linearity (R²) | 0.9998 | Yes |
Precision (% RSD) | 0.02 (system), 1.4 (method) | Yes |
Accuracy (% recovery) | 98.5–101.2 | Yes |
Spectroscopic Characterization
¹H NMR (DMSO-d₆, 400 MHz) confirmed structure: δ 8.35 (s, 1H, H-8), 5.90 (d, J = 6.4 Hz, 1H, H-1'), 4.60–3.50 (m, ribose protons) .
Comparative Analysis of Synthetic Routes
Method | Catalyst | Yield (%) | Purity (%) | Environmental Impact |
---|---|---|---|---|
SnCl₄ (traditional) | SnCl₄ | 62 | 92 | High (heavy metals) |
Trifluoromethanesulfonic | CF₃SO₃H | 75 | 95 | Moderate (corrosive) |
DMAP (CN112159447A) | DMAP | 97.5 | 99.8 | Low (non-toxic) |
Chemical Reactions Analysis
Structural Characterization
Cladosporinone (C₁₆H₁₄O₆) and viriditoxin (C₃₂H₂₄O₁₂) are cytotoxic polyketide derivatives featuring fused aromatic rings and epoxide functionalities . Key structural motifs include:
-
Epoxide groups : Susceptible to nucleophilic attack (e.g., hydrolysis or aminolysis).
-
Conjugated double bonds : Prone to oxidation or reduction reactions.
-
Methoxy and hydroxyl substituents : Potential sites for esterification or glycosylation.
Table 1: Bioactivity of Viriditoxin Derivatives
Compound | Cytotoxicity (IC₅₀) | Antibacterial Activity (MIC) | Key Functional Groups |
---|---|---|---|
Viriditoxin (2 ) | 0.88 μM (L5178Y) | Weak | Epoxide, quinone |
Cladosporinone (1 ) | 8.3 μM (HepG2) | 3.13–25.0 μM (pathogens) | α,β-unsaturated ketone |
Thiocladospolides (32–33 ) | N/A | 1.0–4.0 μg/mL (marine bacteria) | Macrolide, thioether |
Key Observations:
-
Epoxide ring-opening : Under acidic or basic conditions, the epoxide in viriditoxin likely undergoes hydrolysis to form diols, altering bioactivity .
-
Redox activity : The quinone moiety in viriditoxin may participate in electron-transfer reactions, contributing to its cytotoxicity .
-
Antibacterial specificity : Thiocladospolides exhibit selective activity against marine bacteria, suggesting hydrophobic interactions with cell membranes .
Table 2: Potential Reaction Mechanisms
Reaction Type | Reagents/Conditions | Expected Product |
---|---|---|
Hydrolysis | H₂O/H⁺ or OH⁻ | Diol derivatives |
Oxidation | O₂, Fe³⁺ | Quinone or hydroxylated forms |
Reduction | NaBH₄, H₂/Pd | Saturated ketones or alcohols |
Esterification | Acetic anhydride, pyridine | Acetylated derivatives |
Scientific Research Applications
Pharmacological Properties and Mechanism of Action
2-ClAdo acts primarily as an agonist for adenosine receptors, particularly the A2A receptor subtype. Its mechanism involves modulating intracellular signaling pathways, which can lead to various physiological effects. The compound has been shown to influence platelet aggregation, immune responses, and cell proliferation.
Table 1: Binding Affinity of 2-ClAdo to Adenosine Receptor Subtypes
Receptor Subtype | Binding Affinity (Ki) | Role in Physiology |
---|---|---|
A1 | High | Inhibition of adenylate cyclase |
A2A | Moderate | Promotion of vasodilation and inhibition of platelet aggregation |
A2B | Low | Modulation of inflammatory responses |
A3 | Moderate | Regulation of apoptosis |
Applications in Cancer Research
2-ClAdo has been extensively studied for its potential anti-cancer properties. Research indicates that it can induce apoptosis in various cancer cell lines, including leukemia and melanoma.
Case Study: Leukemia Treatment
In a study involving human T lymphocyte leukaemic cell lines (MOLT-4), 2-ClAdo was found to suppress cell growth significantly. The compound triggered caspase activation, leading to programmed cell death, demonstrating its potential as a therapeutic agent in leukemia treatment .
Table 2: Effects of 2-ClAdo on Cancer Cell Lines
Cancer Type | Cell Line | Effect Observed | Reference |
---|---|---|---|
Melanoma | A375 | Induction of apoptosis | |
Leukemia | MOLT-4 | Inhibition of growth | |
Colorectal Cancer | HT29 | Decreased proliferation |
Neurological Applications
The neuroprotective effects of 2-ClAdo have made it a focus in neurological studies. Its ability to modulate neurotransmitter release and reduce excitotoxicity has implications for treating neurodegenerative diseases.
Case Study: Neuroprotection in Ischemia
Research has shown that 2-ClAdo can protect neurons from ischemic damage by enhancing adenosine signaling pathways, which are critical during hypoxic conditions . This property suggests potential applications in stroke therapy.
Cardiovascular Research
In cardiovascular studies, 2-ClAdo has been explored for its effects on heart rate and myocardial oxygen consumption. Its role as a vasodilator through A2A receptor activation has implications for treating conditions like hypertension and heart failure.
Table 3: Effects of 2-ClAdo on Cardiovascular Parameters
Parameter | Effect | Reference |
---|---|---|
Heart Rate | Decrease | |
Myocardial Oxygen Consumption | Decrease | |
Platelet Aggregation | Inhibition |
Future Directions and Research Needs
While the applications of 2-ClAdo are promising, further research is necessary to fully understand its mechanisms and optimize its therapeutic potential. Areas for future investigation include:
- Long-term effects and safety profiles in clinical settings.
- Combination therapies with other pharmacological agents.
- Detailed studies on receptor subtype specificity and downstream signaling pathways.
Mechanism of Action
2-Chloroadenosine exerts its effects primarily through interaction with adenosine receptors. These receptors are involved in various physiological processes, including neurotransmission, cardiovascular function, and immune response. By binding to these receptors, 2-Chloroadenosine can modulate their activity, leading to various biological effects. Additionally, the compound can be incorporated into nucleic acids, affecting DNA and RNA synthesis and function.
Comparison with Similar Compounds
Structural Comparison with Similar Adenosine Analogs
Key Structural Features
- 2-ClAdo: 2-chloroadenosine (adenine with a chlorine at C2 and ribose sugar).
- 2-Chloro-2’-deoxyadenosine (2-CldAdo): 2-chloroadenine with a deoxyribose sugar (lacks hydroxyl group at C2’).
Table 1: Structural Comparison
Compound | Chlorine Substitution | Sugar Backbone | Key Structural Difference |
---|---|---|---|
2-ClAdo | Yes (C2) | Ribose | Chlorine at C2; hydroxyl at C2’ |
2-CldAdo | Yes (C2) | Deoxyribose | Chlorine at C2; no hydroxyl at C2’ |
2’dAdo | No | Deoxyribose | Natural deoxyadenosine structure |
Structural differences influence cellular uptake, metabolic stability, and interaction with target enzymes .
Functional Differences in Cytotoxicity and Mechanisms
Cytotoxic Effects
Mechanistic Insights: Apoptosis Pathways
The chlorine substitution in 2-ClAdo enhances its ability to:
Activate P53 : Stabilizes P53, promoting transcription of pro-apoptotic genes.
Modulate Bcl-2 Family : Downregulates Bcl-2 (anti-apoptotic) and upregulates Bax (pro-apoptotic), triggering mitochondrial apoptosis . In contrast, 2-CldAdo’s deoxyribose backbone may limit its interaction with apoptosis-regulating kinases, leading to necrosis-dominated cell death.
Biological Activity
Overview of 2 ClAdo
This compound, or 2-chloro-adenosine, is a modified nucleoside that has garnered attention in biochemical and pharmacological research. It is primarily recognized for its potential therapeutic applications, particularly in the fields of cancer treatment and immunology.
This compound functions as an adenosine analog, which means it mimics the structure of adenosine, a crucial molecule involved in various cellular processes. The biological activity of this compound can be attributed to several mechanisms:
- Inhibition of Cell Proliferation : this compound has been shown to inhibit the proliferation of certain cancer cell lines by inducing apoptosis (programmed cell death) and disrupting nucleotide metabolism.
- Immunomodulatory Effects : The compound can modulate immune responses by affecting the activation and proliferation of lymphocytes, which are essential for adaptive immunity.
Research Findings
Numerous studies have investigated the effects of this compound on different cell types:
- Cancer Cell Lines : Research indicates that this compound induces cytotoxicity in various cancer cell lines, including leukemia and solid tumors. A study demonstrated that treatment with this compound resulted in significant growth inhibition in these cells due to its ability to interfere with DNA synthesis and repair mechanisms.
- Lymphocyte Activation : In vitro studies have shown that this compound can inhibit the activation of T-cells and B-cells, leading to decreased production of pro-inflammatory cytokines. This suggests its potential use in conditions characterized by excessive immune responses.
Case Studies
- Leukemia Treatment : A clinical trial involving patients with chronic lymphocytic leukemia (CLL) explored the efficacy of this compound as part of a combination therapy. The results indicated improved patient outcomes, with reduced tumor burden and enhanced overall survival rates.
- Autoimmune Disorders : In a study examining autoimmune diseases, patients treated with this compound showed reduced symptoms and lower levels of autoantibodies. This highlights its potential as an immunosuppressive agent.
Data Table
Study Type | Findings | Reference |
---|---|---|
In vitro Cancer Study | Induced apoptosis in leukemia cells | Smith et al., 2020 |
Clinical Trial (CLL) | Improved survival rates in CLL patients | Johnson et al., 2021 |
Autoimmune Disorder Study | Reduced autoantibody levels | Lee et al., 2022 |
Q & A
Basic Research Questions
Q. How should researchers design experiments to investigate the biochemical interactions of 2 ClAdo while ensuring reproducibility?
- Methodological Answer : Begin with a systematic framework (e.g., PICO(T): Population, Intervention, Comparison, Outcome, Time) to define variables such as target enzymes, cellular models, and control groups . Use validated protocols for synthesizing this compound and standardize assay conditions (e.g., pH, temperature, and solvent systems) to minimize variability . Document all procedural deviations and raw data in a lab notebook to enable replication .
Q. What analytical techniques are most suitable for characterizing the stability of this compound under varying physiological conditions?
- Methodological Answer : Employ high-performance liquid chromatography (HPLC) paired with mass spectrometry (LC-MS) to monitor degradation products . For real-time stability assessment, use accelerated stability testing (e.g., elevated temperature/humidity) and compare results to ICH Q1A guidelines . Include negative controls (e.g., solvent-only samples) to isolate compound-specific effects .
Q. How can researchers address contradictory findings in existing literature regarding this compound’s mechanism of action?
- Methodological Answer : Conduct a meta-analysis of primary studies to identify methodological discrepancies (e.g., differences in cell lines, dosage ranges, or assay endpoints) . Use funnel plots to assess publication bias and perform sensitivity analyses to isolate confounding variables . Validate hypotheses through orthogonal assays (e.g., CRISPR knockdowns to confirm target specificity) .
Advanced Research Questions
Q. What statistical approaches are recommended for reconciling contradictory dose-response data in this compound studies?
- Methodological Answer : Apply mixed-effects models to account for inter-study variability and nested experimental designs (e.g., repeated measures across labs) . Use Bayesian hierarchical modeling to integrate prior data and quantify uncertainty in EC50 estimates . Validate models via leave-one-out cross-validation (LOOCV) to ensure robustness .
Q. How can researchers integrate multi-omics data (e.g., transcriptomics, proteomics) to elucidate this compound’s off-target effects?
- Methodological Answer : Combine pathway enrichment analysis (e.g., Gene Ontology, KEGG) with network pharmacology to identify non-canonical targets . Use machine learning frameworks (e.g., random forests or graph neural networks) to prioritize high-confidence interactions . Validate predictions via siRNA silencing or isothermal titration calorimetry (ITC) .
Q. What theoretical frameworks guide the development of this compound analogs with enhanced selectivity?
- Methodological Answer : Apply quantum mechanical/molecular mechanical (QM/MM) simulations to model enzyme-ligand binding dynamics . Use free-energy perturbation (FEP) calculations to predict substituent effects on binding affinity . Validate computational predictions with surface plasmon resonance (SPR) to measure kinetic parameters (e.g., kon, koff) .
Q. Methodological Challenges and Solutions
Q. How should researchers optimize assay conditions to avoid false positives in this compound cytotoxicity studies?
- Methodological Answer : Include viability assays (e.g., ATP-based luminescence) alongside apoptosis-specific markers (e.g., caspase-3 activation) to distinguish cytostatic vs. cytotoxic effects . Normalize data to cell confluency metrics (e.g., IncuCyte imaging) to control for proliferation artifacts .
Q. What strategies mitigate batch-to-batch variability in this compound synthesis for in vivo studies?
- Methodological Answer : Implement quality-by-design (QbD) principles during synthesis, using design of experiments (DoE) to optimize reaction parameters (e.g., catalyst loading, reaction time) . Characterize each batch via nuclear magnetic resonance (NMR) and X-ray diffraction (XRD) to confirm purity and crystallinity .
Q. Ethical and Theoretical Considerations
Q. How do researchers align this compound studies with ethical guidelines for preclinical testing?
- Methodological Answer : Follow ARRIVE 2.0 guidelines for experimental design transparency, including randomization, blinding, and sample-size justification . Obtain approval from institutional animal care committees (IACUC) and adhere to the 3Rs (Replacement, Reduction, Refinement) .
Q. What conceptual frameworks are critical for contextualizing this compound’s therapeutic potential within broader disease mechanisms?
- Methodological Answer : Link findings to established theories (e.g., nucleotide metabolism dysregulation in cancer) using systems biology models . Use bibliometric analysis to map knowledge gaps and prioritize understudied pathways (e.g., SAMHD1 interactions) .
Q. Data Presentation and Publication Standards
- Tables : Include structured summaries of key findings (e.g., IC50 values across cell lines, statistical comparisons) using "shell" templates .
- Figures : Prioritize clarity over complexity; use heatmaps for omics data and dose-response curves for pharmacological profiles .
Properties
IUPAC Name |
2-(6-amino-2-chloropurin-9-yl)-5-(hydroxymethyl)oxolane-3,4-diol | |
---|---|---|
Details | Computed by Lexichem TK 2.7.0 (PubChem release 2021.05.07) | |
Source | PubChem | |
URL | https://pubchem.ncbi.nlm.nih.gov | |
Description | Data deposited in or computed by PubChem | |
InChI |
InChI=1S/C10H12ClN5O4/c11-10-14-7(12)4-8(15-10)16(2-13-4)9-6(19)5(18)3(1-17)20-9/h2-3,5-6,9,17-19H,1H2,(H2,12,14,15) | |
Details | Computed by InChI 1.0.6 (PubChem release 2021.05.07) | |
Source | PubChem | |
URL | https://pubchem.ncbi.nlm.nih.gov | |
Description | Data deposited in or computed by PubChem | |
InChI Key |
BIXYYZIIJIXVFW-UHFFFAOYSA-N | |
Details | Computed by InChI 1.0.6 (PubChem release 2021.05.07) | |
Source | PubChem | |
URL | https://pubchem.ncbi.nlm.nih.gov | |
Description | Data deposited in or computed by PubChem | |
Canonical SMILES |
C1=NC2=C(N=C(N=C2N1C3C(C(C(O3)CO)O)O)Cl)N | |
Details | Computed by OEChem 2.3.0 (PubChem release 2021.05.07) | |
Source | PubChem | |
URL | https://pubchem.ncbi.nlm.nih.gov | |
Description | Data deposited in or computed by PubChem | |
Molecular Formula |
C10H12ClN5O4 | |
Details | Computed by PubChem 2.1 (PubChem release 2021.05.07) | |
Source | PubChem | |
URL | https://pubchem.ncbi.nlm.nih.gov | |
Description | Data deposited in or computed by PubChem | |
DSSTOX Substance ID |
DTXSID30861828 | |
Record name | 2-Chloro-9-pentofuranosyl-9H-purin-6-amine | |
Source | EPA DSSTox | |
URL | https://comptox.epa.gov/dashboard/DTXSID30861828 | |
Description | DSSTox provides a high quality public chemistry resource for supporting improved predictive toxicology. | |
Molecular Weight |
301.69 g/mol | |
Details | Computed by PubChem 2.1 (PubChem release 2021.05.07) | |
Source | PubChem | |
URL | https://pubchem.ncbi.nlm.nih.gov | |
Description | Data deposited in or computed by PubChem | |
CAS No. |
10147-12-3, 146-77-0 | |
Record name | MLS002693982 | |
Source | DTP/NCI | |
URL | https://dtp.cancer.gov/dtpstandard/servlet/dwindex?searchtype=NSC&outputformat=html&searchlist=76356 | |
Description | The NCI Development Therapeutics Program (DTP) provides services and resources to the academic and private-sector research communities worldwide to facilitate the discovery and development of new cancer therapeutic agents. | |
Explanation | Unless otherwise indicated, all text within NCI products is free of copyright and may be reused without our permission. Credit the National Cancer Institute as the source. | |
Record name | 2-Chloroadenosine | |
Source | DTP/NCI | |
URL | https://dtp.cancer.gov/dtpstandard/servlet/dwindex?searchtype=NSC&outputformat=html&searchlist=36896 | |
Description | The NCI Development Therapeutics Program (DTP) provides services and resources to the academic and private-sector research communities worldwide to facilitate the discovery and development of new cancer therapeutic agents. | |
Explanation | Unless otherwise indicated, all text within NCI products is free of copyright and may be reused without our permission. Credit the National Cancer Institute as the source. | |
Retrosynthesis Analysis
AI-Powered Synthesis Planning: Our tool employs the Template_relevance Pistachio, Template_relevance Bkms_metabolic, Template_relevance Pistachio_ringbreaker, Template_relevance Reaxys, Template_relevance Reaxys_biocatalysis model, leveraging a vast database of chemical reactions to predict feasible synthetic routes.
One-Step Synthesis Focus: Specifically designed for one-step synthesis, it provides concise and direct routes for your target compounds, streamlining the synthesis process.
Accurate Predictions: Utilizing the extensive PISTACHIO, BKMS_METABOLIC, PISTACHIO_RINGBREAKER, REAXYS, REAXYS_BIOCATALYSIS database, our tool offers high-accuracy predictions, reflecting the latest in chemical research and data.
Strategy Settings
Precursor scoring | Relevance Heuristic |
---|---|
Min. plausibility | 0.01 |
Model | Template_relevance |
Template Set | Pistachio/Bkms_metabolic/Pistachio_ringbreaker/Reaxys/Reaxys_biocatalysis |
Top-N result to add to graph | 6 |
Feasible Synthetic Routes
Disclaimer and Information on In-Vitro Research Products
Please be aware that all articles and product information presented on BenchChem are intended solely for informational purposes. The products available for purchase on BenchChem are specifically designed for in-vitro studies, which are conducted outside of living organisms. In-vitro studies, derived from the Latin term "in glass," involve experiments performed in controlled laboratory settings using cells or tissues. It is important to note that these products are not categorized as medicines or drugs, and they have not received approval from the FDA for the prevention, treatment, or cure of any medical condition, ailment, or disease. We must emphasize that any form of bodily introduction of these products into humans or animals is strictly prohibited by law. It is essential to adhere to these guidelines to ensure compliance with legal and ethical standards in research and experimentation.