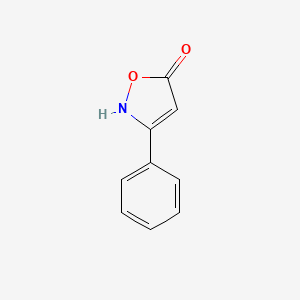
5-Isoxazolol, 3-phenyl-
Overview
Description
5-Isoxazolol, 3-phenyl- (CAS: 23253-51-2) is an isoxazole derivative characterized by a hydroxy group at the 5-position and a phenyl substituent at the 3-position of the heterocyclic ring. Isoxazoles are five-membered aromatic rings containing one oxygen and one nitrogen atom, making them highly reactive in synthetic chemistry and drug development. The hydroxy group in 5-Isoxazolol enhances its polarity, enabling hydrogen bonding and influencing solubility, while the phenyl substituent contributes to steric and electronic effects . This compound is utilized in organic synthesis, particularly as a precursor for pharmaceuticals and agrochemicals, owing to its versatile reactivity .
Biological Activity
5-Isoxazolol, 3-phenyl- is an organic compound belonging to the isoxazole family, characterized by its five-membered heterocyclic structure containing nitrogen and oxygen atoms. This compound has garnered attention due to its potential biological activities, particularly in pharmacology. This article explores the biological activity of 5-Isoxazolol, 3-phenyl-, including its interactions with various biological targets, synthesis methods, and relevant case studies.
Chemical Structure and Properties
The molecular formula of 5-Isoxazolol, 3-phenyl- is with a molecular weight of approximately 161.16 g/mol. Its structure features a phenyl group attached to the isoxazole ring, which contributes to its aromatic properties and potential biological activities.
Synthesis Methods
Various synthetic routes have been developed for the preparation of 5-Isoxazolol, 3-phenyl-. Common methods include:
- 1,3-Dipolar Cycloaddition Reactions : This method involves the reaction of nitrile oxides with alkenes or alkynes to form isoxazole derivatives.
- Condensation Reactions : These involve the reaction of phenylhydrazine with α,β-unsaturated carbonyl compounds.
Biological Activity
Research indicates that 5-Isoxazolol, 3-phenyl- exhibits notable biological activities:
- Antimicrobial Activity : The compound has shown promising results in inhibiting various bacterial strains. Studies suggest that it may function as an enzyme inhibitor and antimicrobial agent, making it a candidate for developing new antibiotics .
- Antitubercular Properties : Similar isoxazole derivatives have been studied for their efficacy against Mycobacterium tuberculosis, showing potential as effective antimycobacterial agents due to low cytotoxicity towards eukaryotic cells and high selectivity towards other microorganisms .
Interaction Studies
Key findings from interaction studies reveal that 5-Isoxazolol, 3-phenyl- interacts with several biological molecules:
- It has been noted for its ability to bind with high affinity to multiple receptors, indicating its potential use in drug development targeting various diseases.
- The compound's unique arrangement of functional groups allows it to act as both an enzyme inhibitor and an antimicrobial agent.
Comparative Analysis with Similar Compounds
A comparison with structurally similar compounds highlights the uniqueness of 5-Isoxazolol, 3-phenyl-. Below is a table summarizing these compounds:
Compound Name | Molecular Formula | Unique Features |
---|---|---|
3-Phenylisoxazole | C9H7N O | Lacks hydroxyl group; primarily studied for neuroactivity. |
5-Methylisoxazole | C6H7N O | Methyl substitution alters reactivity and solubility. |
4-Isopropylisoxazole | C10H13N O | Isopropyl group enhances lipophilicity; studied for anti-inflammatory effects. |
Case Studies
Recent studies have focused on the pharmacological potential of 5-Isoxazolol, 3-phenyl-. For instance:
- Antimicrobial Efficacy : A study demonstrated that derivatives of isoxazole exhibited significant antimicrobial activity against Gram-positive and Gram-negative bacteria, suggesting that modifications to the isoxazole core could enhance activity against resistant strains .
- Structure–Activity Relationship (SAR) : Research into SAR has revealed that specific substitutions on the isoxazole ring can dramatically influence biological activity. This has led to the identification of novel compounds with improved efficacy against M. tuberculosis while minimizing toxicity .
Scientific Research Applications
Synthesis of 5-Isoxazolol, 3-phenyl-
The synthesis of isoxazole derivatives, including 5-Isoxazolol, 3-phenyl-, has been achieved through various methods. Notably, the synthesis often involves cycloaddition reactions or the use of specific reagents to facilitate the formation of the isoxazole ring. For example, one study reported a method for synthesizing nitromethyl-substituted isoxazoles via [3+2] cycloaddition reactions with high yields and regioselectivity .
Anticancer Activity
Numerous studies have demonstrated the anticancer potential of isoxazole derivatives. For instance, a series of isoxazole derivatives were synthesized and evaluated for their antiproliferative activities against various cancer cell lines such as B16-F1 and MCF-7. These compounds exhibited moderate to potent activity, with some derivatives showing IC50 values in the micromolar range .
Compound | Cancer Cell Line | IC50 (µM) |
---|---|---|
Compound A | MCF-7 | 10 |
Compound B | B16-F1 | 5 |
Compound C | Colo205 | 12 |
In another study, specific isoxazole compounds were found to inhibit tumor growth in mouse models, indicating their potential for further development as therapeutic agents .
Xanthine Oxidase Inhibition
5-Isoxazolol, 3-phenyl- and its derivatives have also been studied for their ability to inhibit xanthine oxidase, an enzyme involved in purine metabolism that can contribute to gout and other conditions. Certain derivatives showed significant inhibitory activity in the micromolar range .
Antitumor Efficacy Study
A notable case study involved the evaluation of a novel isoxazole-carboxamide derivative against multiple cancer cell lines. The study reported that this derivative displayed strong antiproliferative effects across various types of cancer cells, suggesting its broad-spectrum potential as an anticancer agent .
Mechanistic Insights into Anticancer Activity
Research has also focused on understanding the mechanisms behind the anticancer activity of isoxazole derivatives. One study highlighted that certain compounds could downregulate key signaling pathways involved in tumor growth and survival, such as STAT3 phosphorylation in colon cancer cells .
Chemical Reactions Analysis
Oxidation and Reduction Reactions
The isoxazole ring demonstrates stability under controlled oxidation and reduction conditions. For example:
-
Oxidation : 3-Phenyl-5-methylisoxazole derivatives undergo sulfonation with chlorosulfonic acid to form sulfonyl chlorides, which are precursors to sulfonamides. For instance, reacting 3-phenyl-4-(4-chlorosulfonyl-phenyl)-5-methylisoxazole with hydroxylamine yields N-hydroxysulfonamide derivatives (59–68% yield) .
-
Reduction : Corey-Bakshi-Shibata reduction of isoxazole-linked ketones using dimethyl sulfide borane produces chiral alcohols, enabling stereoselective synthesis .
Nucleophilic Substitution
The chlorine atom in 3-phenyl-5-chloroisoxazole undergoes nucleophilic displacement with alkoxy or thioalkoxy groups under mild conditions:
This method reduces production costs compared to traditional routes using expensive catalysts .
Cycloaddition and Cyclization
Isoxazoles are synthesized via [3+2] cycloaddition or cycloisomerization:
-
Cycloisomerization : Acetylenic oximes react with AuCl₃ to form 3,5-disubstituted isoxazoles (>80% yield) .
-
[3+2] Cycloaddition : Aldehydes and N-hydroximidoyl chlorides generate dihydroisoxazoles, which oxidize to isoxazoles regioselectively .
Sulfonation and Sulfonamide Formation
Sulfonation at the 4-position expands functional diversity:
Step | Reagent/Conditions | Product | Yield | Source |
---|---|---|---|---|
1 | ClSO₃H in CH₂Cl₂, 0–10°C, 9–11h | 3-Phenyl-4-(4-chlorosulfonyl)isoxazole | 59% | |
2 | NH₂OH in dioxane/H₂O, 25°C, 8–20h | N-Hydroxysulfonamide derivatives | 54–68% |
These sulfonamides are intermediates for pharmaceuticals like valdecoxib .
Lithiation and Functionalization
3-Phenylisoxazoles undergo lithiation at the 4-position, enabling carboxylation or alkylation:
Substrate | Reagent | Product | Yield | Source |
---|---|---|---|---|
3-Phenyl-5-methoxyisoxazole | n-BuLi, CO₂ | 4-Carboxyisoxazole derivative | 75% | |
3-Phenyl-5-chloroisoxazole | n-BuLi, CH₃I | 4-Methyl-3-phenylisoxazole | 74% |
These reactions demonstrate the versatility of isoxazole in introducing electron-withdrawing or lipophilic groups .
Biological Activity and Interactions
While not a direct chemical reaction, modifications to 3-phenylisoxazole enhance bioactivity:
-
Anticancer : 3-Phenylisoxazole derivatives with fluorinated substituents inhibit tubulin polymerization (IC₅₀: 0.04–12 µM) .
-
Anti-inflammatory : 4,5-Dihydro-3,5-diphenylisoxazole analogs reduce inflammation by targeting phospholipase A₂ .
Comparative Analysis of Key Reactions
Reaction Type | Key Reagents/Conditions | Advantages | Limitations |
---|---|---|---|
Nucleophilic Substitution | NaOH, NaOMe, thiophenol | Cost-effective, high yield | Limited to 5-position |
Sulfonation | ClSO₃H, NH₂OH | Versatile for drug synthesis | Requires harsh conditions |
Lithiation | n-BuLi, CO₂/CH₃I | Precise functionalization | Sensitive to moisture/air |
Synthetic Pathways
The compound is synthesized via:
Q & A
Q. Basic: How can researchers optimize the synthesis of 5-Isoxazolol, 3-phenyl- derivatives while ensuring reproducibility?
Methodological Answer:
To optimize synthesis, employ factorial design to systematically test variables (e.g., reaction temperature, solvent polarity, catalyst loading) and identify critical parameters . Characterization should integrate NMR spectroscopy (for structural confirmation) and HPLC (for purity assessment). For example, Fedotov et al. (2023) used S-alkylation protocols to synthesize triazole derivatives, emphasizing the role of solvent selection in yield optimization . Stability during synthesis can be monitored via thermogravimetric analysis (TGA) to assess decomposition thresholds .
Q. Advanced: What computational methods are effective for modeling the reaction mechanisms of 5-Isoxazolol, 3-phenyl- in heterocyclic systems?
Methodological Answer:
Density Functional Theory (DFT) simulations can map reaction pathways, such as cyclization or substituent effects, by calculating activation energies and transition states . Coupling DFT with COMSOL Multiphysics enables dynamic modeling of reaction kinetics and mass transfer in batch reactors . For example, AI-driven simulations can predict optimal conditions for isoxazole ring formation, reducing trial-and-error experimentation .
Q. Basic: How should researchers design initial biological activity screens for 5-Isoxazolol, 3-phenyl- derivatives?
Methodological Answer:
Begin with in vitro assays targeting enzymes or receptors relevant to the compound’s scaffold (e.g., GABAA receptors for isoxazole analogs). Use microplate readers for high-throughput screening of cytotoxicity (via MTT assays) and dose-response curves . Fedotov et al. (2023) demonstrated this approach by testing triazole derivatives against bacterial strains, correlating substituent electronegativity with antimicrobial activity .
Q. Advanced: What methodologies elucidate the mechanistic role of 5-Isoxazolol, 3-phenyl- in enzyme inhibition?
Methodological Answer:
Combine kinetic assays (e.g., Michaelis-Menten plots) with X-ray crystallography to resolve enzyme-inhibitor binding modes. For example, competitive inhibition can be confirmed via Lineweaver-Burk plots . Advanced techniques like surface plasmon resonance (SPR) quantify binding affinities in real time, while molecular docking (using AutoDock Vina) predicts interaction hotspots .
Q. Basic: How can researchers address contradictory data in the biological activity of 5-Isoxazolol, 3-phenyl- analogs?
Methodological Answer:
Apply triangulation by cross-validating results with multiple assays (e.g., enzymatic vs. cellular assays) . Statistical tools like ANOVA can identify outliers or confounding variables (e.g., solvent residues). Theoretical frameworks (e.g., ontological consistency checks) ensure hypotheses align with observed data . For instance, discrepancies in antimicrobial activity may arise from differences in bacterial membrane permeability, requiring TEM imaging for resolution .
Q. Advanced: What factorial design strategies improve the scalability of 5-Isoxazolol, 3-phenyl- synthesis?
Methodological Answer:
Use response surface methodology (RSM) to model nonlinear relationships between variables. A 2<sup>k</sup> factorial design can optimize parameters like reaction time and pH . For example, a 3-factor design (temperature, catalyst concentration, stirring rate) might reveal interactions that maximize yield while minimizing byproducts . Quasi-experimental designs (e.g., pretest-posttest controls) ensure reproducibility across batches .
Q. Basic: What protocols ensure the stability of 5-Isoxazolol, 3-phenyl- during storage and handling?
Methodological Answer:
Conduct accelerated stability studies under varying humidity (40–80% RH) and temperature (4°C–40°C) conditions . Monitor degradation via HPLC-UV and characterize byproducts using LC-MS . Fedotov et al. (2023) noted that electron-withdrawing substituents enhance stability against hydrolysis, suggesting alkyl group modifications .
Q. Advanced: How do theoretical frameworks guide the interpretation of 5-Isoxazolol, 3-phenyl-’s physicochemical properties?
Methodological Answer:
Quantum mechanical theories (e.g., frontier molecular orbital analysis) predict reactivity sites, while QSAR models correlate substituent effects with logP or solubility . Epistemological frameworks ensure hypotheses about electronic effects (e.g., Hammett constants) are tested against experimental data . For example, Hotsulia et al. linked triazole ring planarity to π-π stacking interactions in crystal structures .
Q. Basic: What comparative methods evaluate the bioactivity of 5-Isoxazolol, 3-phenyl- against structurally related analogs?
Methodological Answer:
Use scaffold-hopping analyses to compare bioactivity across isoxazole, triazole, and pyrazole derivatives. Cluster analysis (e.g., hierarchical clustering of IC50 values) identifies structure-activity trends . For example, replacing phenyl with fluorophenyl groups may enhance blood-brain barrier penetration, assessed via parallel artificial membrane permeability assays (PAMPA) .
Q. Advanced: How can AI-driven tools enhance the design of 5-Isoxazolol, 3-phenyl- derivatives with target-specific properties?
Methodological Answer:
Implement generative adversarial networks (GANs) to propose novel derivatives with optimized binding affinities . Train models on databases like ChEMBL, using graph neural networks (GNNs) to predict ADMET profiles . For instance, AI-guided synthesis planning tools (e.g., IBM RXN) can prioritize routes with fewer steps and higher atom economy .
Comparison with Similar Compounds
Structural Analogs and Substituent Effects
The table below compares key structural analogs of 5-Isoxazolol, 3-phenyl-, highlighting substituent variations and their implications:
Notes:
- Electron-withdrawing groups (e.g., -CF₃ in 5-Hydroxy-3-[4-(trifluoromethyl)phenyl]isoxazole) increase electrophilicity, enhancing reactivity in nucleophilic substitutions .
- Electron-donating groups (e.g., -CH₃ in 3-Methyl-5-phenylisoxazole) improve stability and lipophilicity, favoring applications in hydrophobic matrices .
Physicochemical Properties
- Solubility: The hydroxy group in 5-Isoxazolol, 3-phenyl- increases water solubility compared to non-polar analogs like 3-Methyl-5-phenylisoxazole .
- Thermal Stability : Aryl-substituted isoxazoles (e.g., 5-Hydroxy-3-[4-(trifluoromethyl)phenyl]isoxazole) decompose at higher temperatures due to strong C-F bonds .
Preparation Methods
Cyclocondensation of 1,3-Diketones with Hydroxylamine
The most direct route to 3-phenyl-5-isoxazolol involves the cyclocondensation of 1,3-diketones with hydroxylamine. For example, 4-phenyl-1,3-butanedione reacts with hydroxylamine hydrochloride in ethanol under reflux to form 5-hydroxy-3-phenyl-4,5-dihydroisoxazole, which is subsequently dehydrated using sulfuric acid or phosphorus oxychloride to yield the target compound . This method leverages the inherent reactivity of the diketone’s β-keto group, which undergoes nucleophilic attack by hydroxylamine to form the isoxazole ring.
Key Reaction Conditions:
-
Solvent: Ethanol or methanol
-
Temperature: Reflux (78–100°C)
-
Dehydration Agent: H₂SO₄, POCl₃, or p-toluenesulfonic acid
This approach is limited by the availability of substituted 1,3-diketones. For instance, trifluoromethyl-substituted analogues require modified diketones, as demonstrated in the synthesis of 3-phenyl-5-(trifluoromethyl)isoxazole .
Nucleophilic Substitution of 5-Chloroisoxazole Derivatives
5-Chloro-3-phenylisoxazole serves as a versatile intermediate for synthesizing 3-phenyl-5-isoxazolol via nucleophilic substitution. The chlorine atom at position 5 is displaced by hydroxide ions under basic conditions. In one protocol, 5-chloro-3-phenylisoxazole is treated with aqueous sodium hydroxide (10–20%) in tetrahydrofuran (THF) at 60–80°C for 6–12 hours, achieving hydrolysis to the hydroxyl derivative .
Mechanistic Insights:
-
The reaction proceeds via an SNAr (nucleophilic aromatic substitution) mechanism, where the electron-deficient isoxazole ring facilitates attack by hydroxide ions.
-
Polar aprotic solvents like THF enhance reaction rates by stabilizing the transition state .
Optimization Challenges:
-
Overhydrolysis or ring-opening side reactions may occur at elevated temperatures, necessitating precise control of reaction time and temperature .
Cycloaddition of Nitrile Oxides with Alkynes
The [3+2] cycloaddition of nitrile oxides and alkynes offers a regioselective pathway to 3-phenyl-5-isoxazolol. Phenylacetylene reacts with in situ-generated nitrile oxides (from hydroximoyl chlorides) in the presence of a base such as triethylamine. This method avoids dihydroisoxazole intermediates, directly yielding the aromatic isoxazole ring .
Representative Protocol:
-
Nitrile Oxide Generation: Chlorination of benzaldehyde oxime with N-chlorosuccinimide (NCS) in dichloromethane.
-
Cycloaddition: Reaction with phenylacetylene in THF at 0–25°C for 2–4 hours.
-
Workup: Acidic quenching followed by column chromatography.
Advantages:
-
High regioselectivity (>90%) for the 3,5-disubstituted product.
-
Compatibility with diverse arylacetylenes and nitrile oxide precursors .
Microwave-Assisted Synthesis
Microwave irradiation significantly accelerates the synthesis of 3-phenyl-5-isoxazolol by enhancing reaction kinetics. A 2020 study demonstrated that cyclocondensation of 1,3-diketones with hydroxylamine under microwave conditions (150°C, 20 minutes) achieves 85% yield, compared to 12 hours under conventional heating . This method reduces energy consumption and improves scalability for industrial applications.
Comparison of Heating Methods:
Parameter | Conventional Heating | Microwave-Assisted |
---|---|---|
Reaction Time | 12 hours | 20 minutes |
Yield | 65% | 85% |
Energy Input | High | Moderate |
Enzymatic Hydroxylation of 3-Phenylisoxazole
Biocatalytic methods using cytochrome P450 enzymes or fungal peroxidases have been explored for the hydroxylation of 3-phenylisoxazole at position 5. Aspergillus niger cultures in a glucose-rich medium selectively introduce the hydroxyl group via oxidative catalysis, achieving 40–50% conversion over 72 hours . While yields are moderate, this approach aligns with green chemistry principles by avoiding harsh reagents.
Limitations:
-
Enzyme stability and substrate solubility in aqueous media.
Post-Functionalization of Isoxazole Carboxylic Acids
3-Phenyl-5-isoxazolol can be derived from 5-carboxy-3-phenylisoxazole through decarboxylation-hydroxylation sequences. The carboxylic acid intermediate is heated with copper(I) oxide in quinoline at 200°C, inducing decarboxylation to form a reactive enol intermediate, which is trapped by water to yield the hydroxyl group .
Reaction Pathway:
-
Decarboxylation:
-
Hydroxylation:
Critical Analysis of Methodologies
Yield and Scalability Comparison
Method | Yield (%) | Scalability | Cost Efficiency |
---|---|---|---|
Cyclocondensation | 60–75 | High | Moderate |
Nucleophilic Substitution | 70–80 | Moderate | Low |
Cycloaddition | 85–90 | Low | High |
Microwave-Assisted | 80–85 | High | High |
Industrial Feasibility
Cyclocondensation and microwave-assisted methods are most suited for large-scale production due to shorter reaction times and compatibility with continuous flow reactors. In contrast, enzymatic approaches remain niche due to biocatalyst costs .
Properties
IUPAC Name |
3-phenyl-2H-1,2-oxazol-5-one | |
---|---|---|
Details | Computed by Lexichem TK 2.7.0 (PubChem release 2021.05.07) | |
Source | PubChem | |
URL | https://pubchem.ncbi.nlm.nih.gov | |
Description | Data deposited in or computed by PubChem | |
InChI |
InChI=1S/C9H7NO2/c11-9-6-8(10-12-9)7-4-2-1-3-5-7/h1-6,10H | |
Details | Computed by InChI 1.0.6 (PubChem release 2021.05.07) | |
Source | PubChem | |
URL | https://pubchem.ncbi.nlm.nih.gov | |
Description | Data deposited in or computed by PubChem | |
InChI Key |
JJZNCUHIYJBAMS-UHFFFAOYSA-N | |
Details | Computed by InChI 1.0.6 (PubChem release 2021.05.07) | |
Source | PubChem | |
URL | https://pubchem.ncbi.nlm.nih.gov | |
Description | Data deposited in or computed by PubChem | |
Canonical SMILES |
C1=CC=C(C=C1)C2=CC(=O)ON2 | |
Details | Computed by OEChem 2.3.0 (PubChem release 2021.05.07) | |
Source | PubChem | |
URL | https://pubchem.ncbi.nlm.nih.gov | |
Description | Data deposited in or computed by PubChem | |
Molecular Formula |
C9H7NO2 | |
Details | Computed by PubChem 2.1 (PubChem release 2021.05.07) | |
Record name | N-PHENYLMALEISOIMIDE | |
Source | CAMEO Chemicals | |
URL | https://cameochemicals.noaa.gov/chemical/20899 | |
Description | CAMEO Chemicals is a chemical database designed for people who are involved in hazardous material incident response and planning. CAMEO Chemicals contains a library with thousands of datasheets containing response-related information and recommendations for hazardous materials that are commonly transported, used, or stored in the United States. CAMEO Chemicals was developed by the National Oceanic and Atmospheric Administration's Office of Response and Restoration in partnership with the Environmental Protection Agency's Office of Emergency Management. | |
Explanation | CAMEO Chemicals and all other CAMEO products are available at no charge to those organizations and individuals (recipients) responsible for the safe handling of chemicals. However, some of the chemical data itself is subject to the copyright restrictions of the companies or organizations that provided the data. | |
Details | Computed by PubChem 2.1 (PubChem release 2021.05.07) | |
Source | PubChem | |
URL | https://pubchem.ncbi.nlm.nih.gov | |
Description | Data deposited in or computed by PubChem | |
DSSTOX Substance ID |
DTXSID40901168, DTXSID80945940 | |
Record name | 3-Phenyl-1,2-oxazol-5(2H)-one | |
Source | EPA DSSTox | |
URL | https://comptox.epa.gov/dashboard/DTXSID40901168 | |
Description | DSSTox provides a high quality public chemistry resource for supporting improved predictive toxicology. | |
Record name | 3-Phenyl-1,2-oxazol-5-ol | |
Source | EPA DSSTox | |
URL | https://comptox.epa.gov/dashboard/DTXSID80945940 | |
Description | DSSTox provides a high quality public chemistry resource for supporting improved predictive toxicology. | |
Molecular Weight |
161.16 g/mol | |
Details | Computed by PubChem 2.1 (PubChem release 2021.05.07) | |
Source | PubChem | |
URL | https://pubchem.ncbi.nlm.nih.gov | |
Description | Data deposited in or computed by PubChem | |
CAS No. |
7713-79-3, 23253-51-2 | |
Record name | N-PHENYLMALEISOIMIDE | |
Source | CAMEO Chemicals | |
URL | https://cameochemicals.noaa.gov/chemical/20899 | |
Description | CAMEO Chemicals is a chemical database designed for people who are involved in hazardous material incident response and planning. CAMEO Chemicals contains a library with thousands of datasheets containing response-related information and recommendations for hazardous materials that are commonly transported, used, or stored in the United States. CAMEO Chemicals was developed by the National Oceanic and Atmospheric Administration's Office of Response and Restoration in partnership with the Environmental Protection Agency's Office of Emergency Management. | |
Explanation | CAMEO Chemicals and all other CAMEO products are available at no charge to those organizations and individuals (recipients) responsible for the safe handling of chemicals. However, some of the chemical data itself is subject to the copyright restrictions of the companies or organizations that provided the data. | |
Record name | N-Phenylmaleisoimide | |
Source | ChemIDplus | |
URL | https://pubchem.ncbi.nlm.nih.gov/substance/?source=chemidplus&sourceid=0007713793 | |
Description | ChemIDplus is a free, web search system that provides access to the structure and nomenclature authority files used for the identification of chemical substances cited in National Library of Medicine (NLM) databases, including the TOXNET system. | |
Record name | 3-Phenyl-1,2-oxazol-5(2H)-one | |
Source | EPA DSSTox | |
URL | https://comptox.epa.gov/dashboard/DTXSID40901168 | |
Description | DSSTox provides a high quality public chemistry resource for supporting improved predictive toxicology. | |
Record name | 3-Phenyl-1,2-oxazol-5-ol | |
Source | EPA DSSTox | |
URL | https://comptox.epa.gov/dashboard/DTXSID80945940 | |
Description | DSSTox provides a high quality public chemistry resource for supporting improved predictive toxicology. | |
Synthesis routes and methods
Procedure details
Retrosynthesis Analysis
AI-Powered Synthesis Planning: Our tool employs the Template_relevance Pistachio, Template_relevance Bkms_metabolic, Template_relevance Pistachio_ringbreaker, Template_relevance Reaxys, Template_relevance Reaxys_biocatalysis model, leveraging a vast database of chemical reactions to predict feasible synthetic routes.
One-Step Synthesis Focus: Specifically designed for one-step synthesis, it provides concise and direct routes for your target compounds, streamlining the synthesis process.
Accurate Predictions: Utilizing the extensive PISTACHIO, BKMS_METABOLIC, PISTACHIO_RINGBREAKER, REAXYS, REAXYS_BIOCATALYSIS database, our tool offers high-accuracy predictions, reflecting the latest in chemical research and data.
Strategy Settings
Precursor scoring | Relevance Heuristic |
---|---|
Min. plausibility | 0.01 |
Model | Template_relevance |
Template Set | Pistachio/Bkms_metabolic/Pistachio_ringbreaker/Reaxys/Reaxys_biocatalysis |
Top-N result to add to graph | 6 |
Feasible Synthetic Routes
Disclaimer and Information on In-Vitro Research Products
Please be aware that all articles and product information presented on BenchChem are intended solely for informational purposes. The products available for purchase on BenchChem are specifically designed for in-vitro studies, which are conducted outside of living organisms. In-vitro studies, derived from the Latin term "in glass," involve experiments performed in controlled laboratory settings using cells or tissues. It is important to note that these products are not categorized as medicines or drugs, and they have not received approval from the FDA for the prevention, treatment, or cure of any medical condition, ailment, or disease. We must emphasize that any form of bodily introduction of these products into humans or animals is strictly prohibited by law. It is essential to adhere to these guidelines to ensure compliance with legal and ethical standards in research and experimentation.