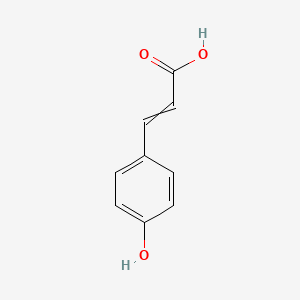
p-Hydroxy-cinnamic acid
Overview
Description
p-Hydroxy-cinnamic acid, also known as cis-p-coumaric acid, is an organic compound with the molecular formula C9H8O3 and a molecular weight of 164.1580 g/mol . This compound is a derivative of cinnamic acid and is characterized by the presence of a hydroxyphenyl group attached to the propenoic acid backbone. It is commonly found in various plants and is known for its antioxidant properties.
Preparation Methods
Synthetic Routes and Reaction Conditions
The synthesis of p-Hydroxy-cinnamic acid can be achieved through several methods. One common approach involves the condensation of 4-hydroxybenzaldehyde with malonic acid in the presence of a base, followed by decarboxylation to yield the desired product . The reaction conditions typically include:
Base: Sodium hydroxide or potassium hydroxide
Solvent: Ethanol or water
Temperature: 60-80°C
Reaction Time: 4-6 hours
Industrial Production Methods
In industrial settings, the production of this compound often involves the use of catalytic processes to enhance yield and efficiency. Catalysts such as palladium on carbon (Pd/C) or nickel can be employed to facilitate the hydrogenation of 4-hydroxycinnamic acid derivatives . The reaction conditions for industrial production may include:
Catalyst: Pd/C or nickel
Solvent: Methanol or ethanol
Temperature: 50-70°C
Pressure: 1-5 atm
Reaction Time: 2-4 hours
Chemical Reactions Analysis
Types of Reactions
p-Hydroxy-cinnamic acid undergoes various chemical reactions, including:
Substitution: The hydroxy group can undergo substitution reactions to form esters or ethers using reagents like acetic anhydride or methyl iodide.
Common Reagents and Conditions
Oxidizing Agents: Potassium permanganate, hydrogen peroxide
Reducing Agents: Sodium borohydride, lithium aluminum hydride
Substitution Reagents: Acetic anhydride, methyl iodide
Major Products Formed
Oxidation: 4-Hydroxybenzoic acid
Reduction: 3-(4-Hydroxyphenyl)propanoic acid
Substitution: Esters or ethers of this compound
Scientific Research Applications
p-Hydroxy-cinnamic acid has a wide range of scientific research applications, including:
Chemistry: Used as a precursor in the synthesis of various organic compounds and polymers.
Biology: Studied for its antioxidant properties and potential role in protecting cells from oxidative stress.
Medicine: Investigated for its anti-inflammatory and anticancer properties.
Industry: Utilized in the production of food additives, cosmetics, and pharmaceuticals.
Mechanism of Action
The mechanism of action of p-Hydroxy-cinnamic acid involves its interaction with various molecular targets and pathways. The compound exerts its effects primarily through:
Antioxidant Activity: Scavenging free radicals and reducing oxidative stress in cells.
Anti-inflammatory Activity: Inhibiting the production of pro-inflammatory cytokines and enzymes.
Anticancer Activity: Inducing apoptosis and inhibiting the proliferation of cancer cells.
Comparison with Similar Compounds
Similar Compounds
p-Coumaric acid, trans: The trans isomer of p-Hydroxy-cinnamic acid, with similar antioxidant properties.
Ferulic acid: A derivative of cinnamic acid with an additional methoxy group, known for its strong antioxidant and anti-inflammatory properties.
Caffeic acid: Another cinnamic acid derivative with two hydroxy groups, widely studied for its antioxidant and anticancer activities.
Uniqueness
This compound is unique due to its specific cis configuration, which can influence its reactivity and interaction with biological targets. This configuration may result in different biological activities compared to its trans isomer and other similar compounds .
Properties
IUPAC Name |
3-(4-hydroxyphenyl)prop-2-enoic acid | |
---|---|---|
Details | Computed by Lexichem TK 2.7.0 (PubChem release 2021.05.07) | |
Source | PubChem | |
URL | https://pubchem.ncbi.nlm.nih.gov | |
Description | Data deposited in or computed by PubChem | |
InChI |
InChI=1S/C9H8O3/c10-8-4-1-7(2-5-8)3-6-9(11)12/h1-6,10H,(H,11,12) | |
Details | Computed by InChI 1.0.6 (PubChem release 2021.05.07) | |
Source | PubChem | |
URL | https://pubchem.ncbi.nlm.nih.gov | |
Description | Data deposited in or computed by PubChem | |
InChI Key |
NGSWKAQJJWESNS-UHFFFAOYSA-N | |
Details | Computed by InChI 1.0.6 (PubChem release 2021.05.07) | |
Source | PubChem | |
URL | https://pubchem.ncbi.nlm.nih.gov | |
Description | Data deposited in or computed by PubChem | |
Canonical SMILES |
C1=CC(=CC=C1C=CC(=O)O)O | |
Details | Computed by OEChem 2.3.0 (PubChem release 2021.05.07) | |
Source | PubChem | |
URL | https://pubchem.ncbi.nlm.nih.gov | |
Description | Data deposited in or computed by PubChem | |
Molecular Formula |
C9H8O3 | |
Details | Computed by PubChem 2.1 (PubChem release 2021.05.07) | |
Source | PubChem | |
URL | https://pubchem.ncbi.nlm.nih.gov | |
Description | Data deposited in or computed by PubChem | |
Related CAS |
50940-26-6 | |
Details | Compound: 2-Propenoic acid, 3-(4-hydroxyphenyl)-, homopolymer | |
Record name | 2-Propenoic acid, 3-(4-hydroxyphenyl)-, homopolymer | |
Source | CAS Common Chemistry | |
URL | https://commonchemistry.cas.org/detail?cas_rn=50940-26-6 | |
Description | CAS Common Chemistry is an open community resource for accessing chemical information. Nearly 500,000 chemical substances from CAS REGISTRY cover areas of community interest, including common and frequently regulated chemicals, and those relevant to high school and undergraduate chemistry classes. This chemical information, curated by our expert scientists, is provided in alignment with our mission as a division of the American Chemical Society. | |
Explanation | The data from CAS Common Chemistry is provided under a CC-BY-NC 4.0 license, unless otherwise stated. | |
DSSTOX Substance ID |
DTXSID6064660 | |
Record name | p-Hydroxycinnamic acid | |
Source | EPA DSSTox | |
URL | https://comptox.epa.gov/dashboard/DTXSID6064660 | |
Description | DSSTox provides a high quality public chemistry resource for supporting improved predictive toxicology. | |
Molecular Weight |
164.16 g/mol | |
Details | Computed by PubChem 2.1 (PubChem release 2021.05.07) | |
Source | PubChem | |
URL | https://pubchem.ncbi.nlm.nih.gov | |
Description | Data deposited in or computed by PubChem | |
CAS No. |
7400-08-0 | |
Record name | p-Coumaric acid | |
Source | CAS Common Chemistry | |
URL | https://commonchemistry.cas.org/detail?cas_rn=7400-08-0 | |
Description | CAS Common Chemistry is an open community resource for accessing chemical information. Nearly 500,000 chemical substances from CAS REGISTRY cover areas of community interest, including common and frequently regulated chemicals, and those relevant to high school and undergraduate chemistry classes. This chemical information, curated by our expert scientists, is provided in alignment with our mission as a division of the American Chemical Society. | |
Explanation | The data from CAS Common Chemistry is provided under a CC-BY-NC 4.0 license, unless otherwise stated. | |
Record name | p-Hydroxycinnamic acid | |
Source | EPA DSSTox | |
URL | https://comptox.epa.gov/dashboard/DTXSID6064660 | |
Description | DSSTox provides a high quality public chemistry resource for supporting improved predictive toxicology. | |
Synthesis routes and methods
Procedure details
Retrosynthesis Analysis
AI-Powered Synthesis Planning: Our tool employs the Template_relevance Pistachio, Template_relevance Bkms_metabolic, Template_relevance Pistachio_ringbreaker, Template_relevance Reaxys, Template_relevance Reaxys_biocatalysis model, leveraging a vast database of chemical reactions to predict feasible synthetic routes.
One-Step Synthesis Focus: Specifically designed for one-step synthesis, it provides concise and direct routes for your target compounds, streamlining the synthesis process.
Accurate Predictions: Utilizing the extensive PISTACHIO, BKMS_METABOLIC, PISTACHIO_RINGBREAKER, REAXYS, REAXYS_BIOCATALYSIS database, our tool offers high-accuracy predictions, reflecting the latest in chemical research and data.
Strategy Settings
Precursor scoring | Relevance Heuristic |
---|---|
Min. plausibility | 0.01 |
Model | Template_relevance |
Template Set | Pistachio/Bkms_metabolic/Pistachio_ringbreaker/Reaxys/Reaxys_biocatalysis |
Top-N result to add to graph | 6 |
Feasible Synthetic Routes
Disclaimer and Information on In-Vitro Research Products
Please be aware that all articles and product information presented on BenchChem are intended solely for informational purposes. The products available for purchase on BenchChem are specifically designed for in-vitro studies, which are conducted outside of living organisms. In-vitro studies, derived from the Latin term "in glass," involve experiments performed in controlled laboratory settings using cells or tissues. It is important to note that these products are not categorized as medicines or drugs, and they have not received approval from the FDA for the prevention, treatment, or cure of any medical condition, ailment, or disease. We must emphasize that any form of bodily introduction of these products into humans or animals is strictly prohibited by law. It is essential to adhere to these guidelines to ensure compliance with legal and ethical standards in research and experimentation.