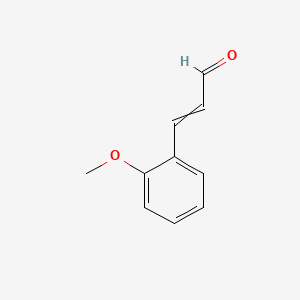
3-(2-Methoxyphenyl)acrylaldehyde
Overview
Description
3-(2-Methoxyphenyl)acrylaldehyde, also known as 2-Methoxycinnamaldehyde, is an organic compound with the molecular formula C10H10O2 and a molecular weight of 162.1852 g/mol . It is a derivative of cinnamaldehyde, characterized by the presence of a methoxy group attached to the phenyl ring. This compound is known for its aromatic properties and is used in various applications, including the synthesis of fragrances and pharmaceuticals .
Preparation Methods
Synthetic Routes and Reaction Conditions
3-(2-Methoxyphenyl)acrylaldehyde can be synthesized through the methylation of salicylaldehyde using dimethyl sulfate. The process involves the following steps :
- Dissolve 3 kg of sodium hydroxide in water to prepare a 30% solution.
- Add the solution containing 12.2 kg of salicylaldehyde and 80 L of water while heating to boiling.
- Slowly add 12.9 kg of dimethyl sulfate, maintaining reflux for approximately 3 hours.
- Continue refluxing for an additional 2-3 hours.
- Cool the mixture, separate the oil layer, and wash with 5% sodium hydroxide solution.
- Wash with water until the pH reaches 8, then dry with anhydrous potassium carbonate.
- Filter and distill under reduced pressure to obtain this compound .
Industrial Production Methods
Industrial production of this compound follows similar synthetic routes but on a larger scale, with optimized reaction conditions to ensure higher yields and purity. The use of continuous flow reactors and advanced purification techniques are common in industrial settings .
Chemical Reactions Analysis
Types of Reactions
3-(2-Methoxyphenyl)acrylaldehyde undergoes various chemical reactions, including:
Oxidation: The aldehyde group can be oxidized to form carboxylic acids.
Reduction: The compound can be reduced to form alcohols.
Substitution: The methoxy group can participate in electrophilic aromatic substitution reactions.
Common Reagents and Conditions
Oxidation: Common oxidizing agents include potassium permanganate (KMnO4) and chromium trioxide (CrO3).
Reduction: Reducing agents such as sodium borohydride (NaBH4) and lithium aluminum hydride (LiAlH4) are used.
Substitution: Electrophilic aromatic substitution reactions often use reagents like bromine (Br2) and nitric acid (HNO3).
Major Products Formed
Oxidation: Formation of 2-Methoxycinnamic acid.
Reduction: Formation of 2-Methoxycinnamyl alcohol.
Substitution: Formation of various substituted derivatives depending on the reagents used.
Scientific Research Applications
3-(2-Methoxyphenyl)acrylaldehyde has diverse applications in scientific research:
Chemistry: Used as an intermediate in organic synthesis and the production of fragrances.
Biology: Studied for its potential antimicrobial and antioxidant properties.
Medicine: Investigated for its potential therapeutic effects, including anti-inflammatory and anticancer activities.
Industry: Utilized in the manufacture of flavoring agents and as a precursor for various chemical compounds
Mechanism of Action
The mechanism of action of 3-(2-Methoxyphenyl)acrylaldehyde involves its interaction with various molecular targets and pathways:
Molecular Targets: The compound can interact with enzymes and receptors, modulating their activity.
Pathways Involved: It may influence signaling pathways related to inflammation, oxidative stress, and cell proliferation.
Comparison with Similar Compounds
Similar Compounds
Cinnamaldehyde: The parent compound, lacking the methoxy group.
2-Methoxycinnamic acid: The oxidized form of 3-(2-Methoxyphenyl)acrylaldehyde.
2-Methoxycinnamyl alcohol: The reduced form of this compound
Uniqueness
This compound is unique due to the presence of the methoxy group, which imparts distinct chemical properties and reactivity compared to its analogs. This functional group enhances its solubility in organic solvents and influences its biological activity .
Properties
IUPAC Name |
3-(2-methoxyphenyl)prop-2-enal | |
---|---|---|
Details | Computed by Lexichem TK 2.7.0 (PubChem release 2021.05.07) | |
Source | PubChem | |
URL | https://pubchem.ncbi.nlm.nih.gov | |
Description | Data deposited in or computed by PubChem | |
InChI |
InChI=1S/C10H10O2/c1-12-10-7-3-2-5-9(10)6-4-8-11/h2-8H,1H3 | |
Details | Computed by InChI 1.0.6 (PubChem release 2021.05.07) | |
Source | PubChem | |
URL | https://pubchem.ncbi.nlm.nih.gov | |
Description | Data deposited in or computed by PubChem | |
InChI Key |
KKVZAVRSVHUSPL-UHFFFAOYSA-N | |
Details | Computed by InChI 1.0.6 (PubChem release 2021.05.07) | |
Source | PubChem | |
URL | https://pubchem.ncbi.nlm.nih.gov | |
Description | Data deposited in or computed by PubChem | |
Canonical SMILES |
COC1=CC=CC=C1C=CC=O | |
Details | Computed by OEChem 2.3.0 (PubChem release 2021.05.07) | |
Source | PubChem | |
URL | https://pubchem.ncbi.nlm.nih.gov | |
Description | Data deposited in or computed by PubChem | |
Molecular Formula |
C10H10O2 | |
Details | Computed by PubChem 2.1 (PubChem release 2021.05.07) | |
Source | PubChem | |
URL | https://pubchem.ncbi.nlm.nih.gov | |
Description | Data deposited in or computed by PubChem | |
DSSTOX Substance ID |
DTXSID2025551 | |
Record name | 2'-Methoxycinnamaldehyde | |
Source | EPA DSSTox | |
URL | https://comptox.epa.gov/dashboard/DTXSID2025551 | |
Description | DSSTox provides a high quality public chemistry resource for supporting improved predictive toxicology. | |
Molecular Weight |
162.18 g/mol | |
Details | Computed by PubChem 2.1 (PubChem release 2021.05.07) | |
Source | PubChem | |
URL | https://pubchem.ncbi.nlm.nih.gov | |
Description | Data deposited in or computed by PubChem | |
CAS No. |
1504-74-1 | |
Record name | 2-Methoxycinnamaldehyde | |
Source | CAS Common Chemistry | |
URL | https://commonchemistry.cas.org/detail?cas_rn=1504-74-1 | |
Description | CAS Common Chemistry is an open community resource for accessing chemical information. Nearly 500,000 chemical substances from CAS REGISTRY cover areas of community interest, including common and frequently regulated chemicals, and those relevant to high school and undergraduate chemistry classes. This chemical information, curated by our expert scientists, is provided in alignment with our mission as a division of the American Chemical Society. | |
Explanation | The data from CAS Common Chemistry is provided under a CC-BY-NC 4.0 license, unless otherwise stated. | |
Record name | 2'-Methoxycinnamaldehyde | |
Source | EPA DSSTox | |
URL | https://comptox.epa.gov/dashboard/DTXSID2025551 | |
Description | DSSTox provides a high quality public chemistry resource for supporting improved predictive toxicology. | |
Retrosynthesis Analysis
AI-Powered Synthesis Planning: Our tool employs the Template_relevance Pistachio, Template_relevance Bkms_metabolic, Template_relevance Pistachio_ringbreaker, Template_relevance Reaxys, Template_relevance Reaxys_biocatalysis model, leveraging a vast database of chemical reactions to predict feasible synthetic routes.
One-Step Synthesis Focus: Specifically designed for one-step synthesis, it provides concise and direct routes for your target compounds, streamlining the synthesis process.
Accurate Predictions: Utilizing the extensive PISTACHIO, BKMS_METABOLIC, PISTACHIO_RINGBREAKER, REAXYS, REAXYS_BIOCATALYSIS database, our tool offers high-accuracy predictions, reflecting the latest in chemical research and data.
Strategy Settings
Precursor scoring | Relevance Heuristic |
---|---|
Min. plausibility | 0.01 |
Model | Template_relevance |
Template Set | Pistachio/Bkms_metabolic/Pistachio_ringbreaker/Reaxys/Reaxys_biocatalysis |
Top-N result to add to graph | 6 |
Feasible Synthetic Routes
Disclaimer and Information on In-Vitro Research Products
Please be aware that all articles and product information presented on BenchChem are intended solely for informational purposes. The products available for purchase on BenchChem are specifically designed for in-vitro studies, which are conducted outside of living organisms. In-vitro studies, derived from the Latin term "in glass," involve experiments performed in controlled laboratory settings using cells or tissues. It is important to note that these products are not categorized as medicines or drugs, and they have not received approval from the FDA for the prevention, treatment, or cure of any medical condition, ailment, or disease. We must emphasize that any form of bodily introduction of these products into humans or animals is strictly prohibited by law. It is essential to adhere to these guidelines to ensure compliance with legal and ethical standards in research and experimentation.