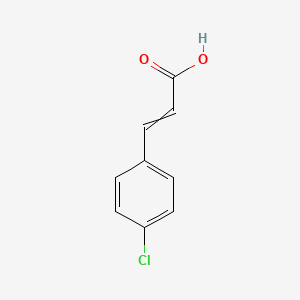
4-CHLOROCINNAMIC ACID
Overview
Description
4-Chlorocinnamic acid (4-CCA; CAS 1615-02-7) is a halogenated derivative of cinnamic acid with the molecular formula C₉H₇ClO₂ and a molecular weight of 182.61 g/mol . Structurally, it consists of a cinnamic acid backbone (a propenoic acid group attached to a benzene ring) with a chlorine substituent at the para position. The compound predominantly exists in the trans-configuration and exhibits a melting point of 246–250°C, a boiling point of 325.3°C, and a density of 1.3 g/cm³ .
4-CCA has garnered attention in materials science due to its photomechanical properties. Thin ribbon-like crystals (≤5 µm) undergo reversible twisting and bending under UV light (365 nm), a response absent in thicker crystals (>10 µm) . This behavior is attributed to [2+2] photodimerization reactions within the crystal lattice . In microbiology, 4-CCA exhibits moderate antimicrobial activity, with MIC values of 708 µM against Escherichia coli and Bacillus subtilis . Derivatives of 4-CCA, particularly esters synthesized via Steglich reactions, show enhanced antibacterial effects against pathogens like Pseudomonas aeruginosa and Staphylococcus aureus .
Preparation Methods
Green Chemistry Synthesis Approach
Solvent-Free Condensation
A solvent-free reaction between 4-chlorobenzaldehyde and malonic acid, catalyzed by piperidine, produces trans-4-chlorocinnamic acid. The absence of organic solvents aligns with green chemistry principles .
Reaction Monitoring and Purification
Thin-layer chromatography (TLC) monitors reaction completion. The mixture is quenched with 5% HCl, extracted with ethyl acetate (3 × 50 mL), and dried over MgSO₄. Column chromatography on silica gel (hexane/ethyl acetate gradient) isolates the pure product, yielding 99% .
Spectroscopic Characterization
-
¹H NMR (300 MHz, CDCl₃) : δ 7.82 (d, J = 15.9 Hz, 1H), 7.65–7.54 (m, 2H), 7.50–7.36 (m, 3H), 6.44 (d, J = 15.9 Hz, 1H) .
-
¹³C NMR (75 MHz, DMSO-d₆) : δ 167.39 (C=O), 142.32 (C-Cl), 136.55–121.03 (aromatic carbons) .
Comparative Analysis of Synthetic Routes
Yield and Purity
Method | Conditions | Yield | Melting Point | Reference |
---|---|---|---|---|
NaOH/NaOCl Oxidation | 50–100°C, aqueous phase | 75% | >248°C | |
Green Chemistry Approach | Solvent-free, column chromatography | 99% | Not reported |
Environmental and Practical Considerations
-
NaOH/NaOCl Method : Uses hazardous hypochlorite but avoids organic solvents.
-
Green Method : High yield and eco-friendly but requires costly chromatography.
Industrial and Scalable Production Insights
Scalability of the NaOH/NaOCl Method
The omission of distillation and use of inexpensive reagents make this method suitable for large-scale production. However, the five-day reaction time may hinder throughput .
Challenges in Green Method Implementation
While the 99% yield is attractive, chromatographic purification is impractical for industrial settings. Alternative purification strategies, such as recrystallization, require exploration .
Analytical Characterization Techniques
Melting Point Analysis
The NaOH/NaOCl method yields a product with a melting point >248°C, consistent with literature values for trans-4-chlorocinnamic acid .
Spectroscopic Validation
NMR data confirm the trans-configuration and purity of the product from both methods. The absence of cis-isomer peaks in the green method’s spectra indicates high stereoselectivity .
Chemical Reactions Analysis
Esterification Reactions
4Cl-CA undergoes esterification via multiple methods to yield bioactive derivatives:
Fischer Esterification
-
Reagents : 4Cl-CA, methanol, sulfuric acid.
-
Conditions : Reflux at 60–80°C for 24–48 hours.
Mitsunobu Reaction
-
Reagents : 4Cl-CA, perillyl alcohol, diisopropyl azodicarboxylate (DIAD), triphenylphosphine.
-
Conditions : Tetrahydrofuran (THF), 0°C to room temperature, 72 hours.
-
Product : Perillyl 4-chlorocinnamate (11 ) with 26.3% yield .
Steglich Esterification
-
Reagents : 4Cl-CA, lauryl alcohol, dicyclohexylcarbodiimide (DCC), 4-dimethylaminopyridine (DMAP).
-
Conditions : Dichloromethane, room temperature, 72 hours.
Table 1: Ester Derivatives of 4Cl-CA
Photochemical [2+2] Cycloaddition
4Cl-CA exhibits solid-state photoreactivity under UV irradiation:
-
Reaction : Irreversible [2+2] photodimerization forming a cyclobutane derivative.
-
Conditions : Crystalline phase, 365 nm UV light, monitored via ¹³C solid-state NMR .
-
Mechanism : Topochemical control aligns molecules for dimerization, with reaction efficiency dependent on crystal size and morphology .
Oxidation of Cinnamyl Alcohol
-
Reagents : trans-4-Chlorocinnamyl alcohol, NaOtBu, oxygen atmosphere.
-
Conditions : Toluene, room temperature.
Catalytic Coupling Reactions
4Cl-CA derivatives are synthesized via diazonium salt intermediates:
-
Reagents : Polyhalogenated anilines, acrylic acid derivatives.
-
Conditions : Diazotization followed by coupling in acidic media .
-
Application : Key intermediates for agrochemicals and pharmaceuticals .
Antimicrobial Activity Correlations
While not a reaction, ester derivatives of 4Cl-CA show structure-dependent bioactivity:
-
Most Potent Antifungal : Perillyl 4-chlorocinnamate (11 , MIC = 0.024 μmol/mL) .
-
Mechanistic Insight : Molecular docking suggests inhibition of fungal 14α-demethylase .
Key Findings and Trends
-
Esterification Efficiency : Methanol and short-chain alcohols yield higher conversions (e.g., 97.6% for methyl ester) .
-
Photoreactivity : Solid-state dimerization is morphology-dependent, enabling potential applications in photomechanical materials .
-
Synthetic Versatility : Boron tribromide-mediated synthesis offers a one-pot route to 4Cl-CA with high purity .
Scientific Research Applications
Antimicrobial Activity
4-Chlorocinnamic acid and its derivatives have demonstrated significant antimicrobial properties, particularly against various strains of bacteria and fungi. Research indicates that esters derived from 4-CCA exhibit enhanced antimicrobial activity compared to the parent compound.
- Study Findings : A study synthesized twelve esters of 4-CCA through various esterification reactions, yielding compounds with diverse antimicrobial efficacy against pathogens such as Staphylococcus aureus, Candida albicans, and Pseudomonas aeruginosa. Notably, methoxyethyl 4-chlorocinnamate and perillyl 4-chlorocinnamate exhibited the most potent antifungal activity with minimum inhibitory concentrations (MIC) of 0.13 and 0.024 μg/mL, respectively .
Compound | MIC (μg/mL) | Target Pathogen |
---|---|---|
Methoxyethyl 4-chlorocinnamate | 0.13 | Candida albicans |
Perillyl 4-chlorocinnamate | 0.024 | Candida albicans |
Methyl 4-chlorocinnamate | Variable | Staphylococcus aureus |
Antioxidant Properties
Research suggests that 4-CCA may possess antioxidant properties, which can help protect cells from oxidative stress caused by free radicals. This property is crucial in preventing chronic diseases linked to oxidative damage .
Anti-inflammatory Effects
In vitro studies indicate that 4-CCA exhibits anti-inflammatory effects, which could have therapeutic implications in treating inflammatory diseases. However, further research is required to elucidate the specific mechanisms involved .
Antitumor Potential
Preliminary studies suggest that 4-CCA may have antitumor properties, although this area of research is still nascent. Investigations into its effectiveness and mechanisms are ongoing .
Molecular Docking Studies
Molecular docking studies have shown that derivatives of 4-CCA can effectively bind to enzymes involved in fungal resistance, such as 14-demethylase. This binding enhances their antifungal efficacy and underscores the importance of structure-activity relationships in drug design .
Case Study: Antimicrobial Efficacy
A comprehensive study focused on synthesizing and testing various esters derived from 4-CCA revealed significant antimicrobial activity against clinically relevant pathogens. The study utilized molecular docking to explore the interaction between these compounds and target enzymes involved in microbial resistance.
Methodology
- Twelve esters were synthesized through Fischer esterification and other methods.
- Antimicrobial tests were conducted against strains including S. aureus and C. albicans.
- Molecular docking studies were performed using the enzyme 14-demethylase.
Results
The results indicated that certain esters not only inhibited microbial growth effectively but also showed promise for further development into therapeutic agents aimed at combating antibiotic-resistant infections.
Case Study: Structure-Activity Relationship (SAR)
An SAR study compared the activity of trans-cinnamic acid derivatives with that of 4-CCA derivatives, revealing that modifications at specific positions significantly influenced their biological activity.
Findings
Mechanism of Action
The mechanism of action of 4-chlorocinnamic acid involves its interaction with molecular targets such as enzymes. For example, esters derived from this compound have been shown to inhibit the enzyme 14-demethylase, which is crucial for antifungal activity . The presence of electron-withdrawing groups on the phenyl ring enhances its biological efficacy .
Comparison with Similar Compounds
Structural Analogs and Physicochemical Properties
Key structural analogs include halogenated, methoxylated, and nitrated cinnamic acid derivatives. Their properties are summarized below:
Antimicrobial Activity
- 4-Methoxycinnamic acid outperforms 4-CCA, with MIC values 10–14× lower against fungi and bacteria (e.g., 50.4 µM vs. Aspergillus niger) .
- aureus at tested concentrations .
- 4-CCA derivatives : Esters synthesized via Steglich reactions exhibit enhanced activity , though specific MIC data are pending .
Photomechanical Behavior
- 4-CCA : Thin crystals (<5 µm) bend reversibly via UV-induced dimerization, while thicker crystals remain inert .
- 4-Fluoro-9-anthracene carboxylic acid : Branched microcrystals demonstrate synchronized bending due to F···H–C interactions, enabling multi-arm actuation .
- 3,5-Dichlorophenyl chalcone : Polymorphic crystals exhibit dual thermal and photomechanical bending, highlighting the role of halogen positioning .
Biological Activity
4-Chlorocinnamic acid (4-CCA) is a derivative of cinnamic acid that has garnered attention for its diverse biological activities, particularly in antimicrobial and anticancer applications. This article explores the biological activity of 4-CCA, summarizing recent research findings, case studies, and relevant data tables.
Antimicrobial Activity
1. Bacterial and Fungal Inhibition
This compound exhibits significant antimicrobial properties against various bacterial and fungal strains. A study synthesized a series of this compound derivatives, which were tested against strains such as Staphylococcus aureus, Pseudomonas aeruginosa, and several Candida species. The results indicated that certain esters derived from 4-CCA demonstrated potent activity, with minimum inhibitory concentrations (MIC) as low as 0.024 μg/mL against Candida albicans .
Compound | MIC (μg/mL) | Target Organism |
---|---|---|
Methoxyethyl 4-chlorocinnamate | 0.13 | Candida albicans |
Perillyl 4-chlorocinnamate | 0.024 | Candida albicans |
Methyl 4-chlorocinnamate | Highest tested concentration | Staphylococcus aureus |
2. Mechanism of Action
Molecular docking studies suggest that the antifungal action of 4-CCA derivatives may involve inhibition of the enzyme 14α-demethylase, a critical enzyme in the biosynthesis of ergosterol in fungi . This mechanism highlights the potential of these compounds as effective antifungal agents.
Cytotoxicity and Anticancer Properties
Research has also investigated the cytotoxic effects of 4-CCA on various cancer cell lines. In vitro studies have shown that certain derivatives exhibit selective cytotoxicity towards cancer cells while maintaining low toxicity to normal cells. For instance, some derivatives demonstrated submicromolar activity against cancer cell lines with minimal impact on primary porcine monocyte-derived macrophages .
Case Studies
Case Study: Antimicrobial Efficacy Against Mycobacteria
A significant study evaluated the efficacy of 4-CCA derivatives against mycobacterial strains, including Mycobacterium tuberculosis. The results indicated that several synthesized compounds exhibited higher efficacy than traditional antibiotics like ampicillin and isoniazid, particularly against resistant strains .
Case Study: Plant Pathogen Inhibition
Another study focused on the application of 4-CCA in agriculture, where it was isolated from Streptomyces griseocarneus. The compound was shown to inhibit the growth of plant pathogens such as Colletotrichum gloeosporioides, reducing lesion sizes significantly in treated plants .
Structure-Activity Relationship (SAR)
The biological activity of 4-CCA is influenced by its chemical structure. Modifications to the core structure can enhance its antimicrobial properties. For example, halogen substitutions have been shown to increase antibacterial activity compared to unsubstituted cinnamic acid derivatives .
Q & A
Basic Research Questions
Q. What are the key physicochemical properties of 4-chlorocinnamic acid, and how do they influence its handling in laboratory settings?
- Answer : Key properties include a molecular weight of 182.61 g/mol, melting point of 248–250°C (lit.), and density of 1.3±0.1 g/cm³ . These properties dictate storage conditions (e.g., desiccated environments to prevent hygroscopic degradation) and handling precautions (e.g., avoiding prolonged exposure to heat due to its high melting point). For purity verification, measure melting point consistency and compare to literature values .
Q. How can researchers validate the identity and purity of synthesized this compound?
- Answer : Use a combination of analytical techniques:
- Melting Point Analysis : Compare observed values (248–250°C) with literature data .
- Spectroscopy : Employ H/C NMR to confirm the trans-configuration (predominant isomer) and FT-IR to identify carboxylic acid and C-Cl functional groups .
- Chromatography : HPLC or TLC with standards to assess purity .
Q. What are the standard synthetic routes for this compound, and what are their limitations?
- Answer : Common methods include:
- Perkin Reaction : Condensation of 4-chlorobenzaldehyde with acetic anhydride in the presence of a base. Limitations include moderate yields (~50–60%) and byproduct formation .
- Knoevenagel Condensation : Requires substituted benzaldehyde and malonic acid. Advantages include higher regioselectivity but may need optimized catalysts .
- Validate synthetic routes using Reaxys or SciFinder to compare reported procedures .
Advanced Research Questions
Q. How can researchers resolve contradictions in reported spectroscopic data for this compound?
- Answer : Discrepancies in NMR/IR data (e.g., peak splitting or shifts) often arise from solvent effects, isomer ratios, or impurities. To address this:
- Replicate experiments under standardized conditions (solvent, temperature).
- Cross-reference data with multiple literature sources (prioritizing peer-reviewed journals over vendor catalogs) .
- Perform computational modeling (e.g., DFT calculations) to predict spectral profiles and compare with empirical results .
Q. What experimental strategies optimize the yield and selectivity of this compound in solvent-free or green synthesis?
- Answer :
- Catalyst Screening : Test ionic liquids or biocatalysts to enhance reaction efficiency.
- Microwave-Assisted Synthesis : Reduces reaction time and improves yield by 15–20% compared to conventional heating .
- Solvent Selection : Use water or ethanol as greener alternatives; monitor polarity effects on isomer ratios .
- Document trial experiments in supplementary materials to support reproducibility claims .
Q. How should researchers design experiments to investigate the structure-activity relationship (SAR) of this compound derivatives?
- Answer :
- Variable Control : Systematically modify substituents (e.g., halogen position, esterification) while keeping reaction conditions constant.
- Bioactivity Assays : Pair synthetic data with in vitro/in silico studies (e.g., enzyme inhibition assays).
- Statistical Analysis : Use ANOVA to identify significant correlations between structural features and activity .
Q. What methodologies are recommended for analyzing degradation products or stability of this compound under varying pH/temperature?
- Answer :
- Accelerated Stability Studies : Expose samples to stress conditions (e.g., 40°C/75% RH) and analyze degradation via LC-MS.
- Kinetic Modeling : Determine half-life and degradation pathways using Arrhenius equations .
- Comparative Studies : Contrast thermal behavior (DSC/TGA) with parent compounds like cinnamic acid .
Q. Data Management and Reproducibility
Q. How can researchers ensure reproducibility when reporting synthetic procedures for this compound?
- Answer :
- Detailed Documentation : Specify reactant ratios, catalyst loadings, and purification steps (e.g., recrystallization solvents).
- Raw Data Inclusion : Provide NMR/IR spectra in supplementary materials with baseline correction annotations .
- Independent Validation : Collaborate with third-party labs to replicate key findings .
Q. What statistical approaches are suitable for resolving discrepancies in biological activity data across studies?
- Answer :
- Meta-Analysis : Aggregate data from multiple studies to identify trends or outliers.
- Error Analysis : Calculate confidence intervals and standard deviations to assess data reliability .
- Machine Learning : Train models to predict bioactivity based on physicochemical descriptors, highlighting conflicting data points for re-evaluation .
Properties
IUPAC Name |
3-(4-chlorophenyl)prop-2-enoic acid | |
---|---|---|
Details | Computed by Lexichem TK 2.7.0 (PubChem release 2021.05.07) | |
Source | PubChem | |
URL | https://pubchem.ncbi.nlm.nih.gov | |
Description | Data deposited in or computed by PubChem | |
InChI |
InChI=1S/C9H7ClO2/c10-8-4-1-7(2-5-8)3-6-9(11)12/h1-6H,(H,11,12) | |
Details | Computed by InChI 1.0.6 (PubChem release 2021.05.07) | |
Source | PubChem | |
URL | https://pubchem.ncbi.nlm.nih.gov | |
Description | Data deposited in or computed by PubChem | |
InChI Key |
GXLIFJYFGMHYDY-UHFFFAOYSA-N | |
Details | Computed by InChI 1.0.6 (PubChem release 2021.05.07) | |
Source | PubChem | |
URL | https://pubchem.ncbi.nlm.nih.gov | |
Description | Data deposited in or computed by PubChem | |
Canonical SMILES |
C1=CC(=CC=C1C=CC(=O)O)Cl | |
Details | Computed by OEChem 2.3.0 (PubChem release 2021.05.07) | |
Source | PubChem | |
URL | https://pubchem.ncbi.nlm.nih.gov | |
Description | Data deposited in or computed by PubChem | |
Molecular Formula |
C9H7ClO2 | |
Details | Computed by PubChem 2.1 (PubChem release 2021.05.07) | |
Source | PubChem | |
URL | https://pubchem.ncbi.nlm.nih.gov | |
Description | Data deposited in or computed by PubChem | |
DSSTOX Substance ID |
DTXSID3075098 | |
Record name | 2-Propenoic acid, 3-(4-chlorophenyl)- | |
Source | EPA DSSTox | |
URL | https://comptox.epa.gov/dashboard/DTXSID3075098 | |
Description | DSSTox provides a high quality public chemistry resource for supporting improved predictive toxicology. | |
Molecular Weight |
182.60 g/mol | |
Details | Computed by PubChem 2.1 (PubChem release 2021.05.07) | |
Source | PubChem | |
URL | https://pubchem.ncbi.nlm.nih.gov | |
Description | Data deposited in or computed by PubChem | |
CAS No. |
1615-02-7 | |
Record name | 4-Chlorocinnamic acid | |
Source | CAS Common Chemistry | |
URL | https://commonchemistry.cas.org/detail?cas_rn=1615-02-7 | |
Description | CAS Common Chemistry is an open community resource for accessing chemical information. Nearly 500,000 chemical substances from CAS REGISTRY cover areas of community interest, including common and frequently regulated chemicals, and those relevant to high school and undergraduate chemistry classes. This chemical information, curated by our expert scientists, is provided in alignment with our mission as a division of the American Chemical Society. | |
Explanation | The data from CAS Common Chemistry is provided under a CC-BY-NC 4.0 license, unless otherwise stated. | |
Record name | 2-Propenoic acid, 3-(4-chlorophenyl)- | |
Source | EPA DSSTox | |
URL | https://comptox.epa.gov/dashboard/DTXSID3075098 | |
Description | DSSTox provides a high quality public chemistry resource for supporting improved predictive toxicology. | |
Retrosynthesis Analysis
AI-Powered Synthesis Planning: Our tool employs the Template_relevance Pistachio, Template_relevance Bkms_metabolic, Template_relevance Pistachio_ringbreaker, Template_relevance Reaxys, Template_relevance Reaxys_biocatalysis model, leveraging a vast database of chemical reactions to predict feasible synthetic routes.
One-Step Synthesis Focus: Specifically designed for one-step synthesis, it provides concise and direct routes for your target compounds, streamlining the synthesis process.
Accurate Predictions: Utilizing the extensive PISTACHIO, BKMS_METABOLIC, PISTACHIO_RINGBREAKER, REAXYS, REAXYS_BIOCATALYSIS database, our tool offers high-accuracy predictions, reflecting the latest in chemical research and data.
Strategy Settings
Precursor scoring | Relevance Heuristic |
---|---|
Min. plausibility | 0.01 |
Model | Template_relevance |
Template Set | Pistachio/Bkms_metabolic/Pistachio_ringbreaker/Reaxys/Reaxys_biocatalysis |
Top-N result to add to graph | 6 |
Feasible Synthetic Routes
Disclaimer and Information on In-Vitro Research Products
Please be aware that all articles and product information presented on BenchChem are intended solely for informational purposes. The products available for purchase on BenchChem are specifically designed for in-vitro studies, which are conducted outside of living organisms. In-vitro studies, derived from the Latin term "in glass," involve experiments performed in controlled laboratory settings using cells or tissues. It is important to note that these products are not categorized as medicines or drugs, and they have not received approval from the FDA for the prevention, treatment, or cure of any medical condition, ailment, or disease. We must emphasize that any form of bodily introduction of these products into humans or animals is strictly prohibited by law. It is essential to adhere to these guidelines to ensure compliance with legal and ethical standards in research and experimentation.