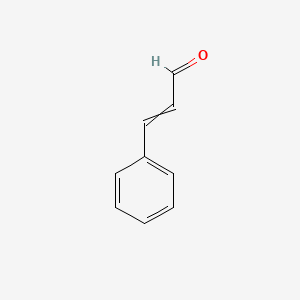
3-Phenyl-2-propenal
Overview
Description
3-Phenyl-2-propenal, also known as cinnamaldehyde, is an organic compound with the molecular formula C9H8O. It is a yellowish, viscous liquid with a distinctive cinnamon odor. This compound is naturally found in the bark of cinnamon trees and other species of the genus Cinnamomum. It is widely used as a flavoring agent, fragrance, and in various industrial applications .
Preparation Methods
Synthetic Routes and Reaction Conditions
3-Phenyl-2-propenal can be synthesized through several methods:
Perkin Reaction: This classical method involves the condensation of benzaldehyde with acetic anhydride in the presence of sodium acetate. The reaction proceeds through the formation of an intermediate, which undergoes dehydration to yield this compound.
Aldol Condensation: Another common method is the aldol condensation of benzaldehyde with acetaldehyde in the presence of a base such as sodium hydroxide. The intermediate aldol product is then dehydrated to form this compound.
Industrial Production Methods
In industrial settings, this compound is typically produced by the catalytic dehydrogenation of cinnamyl alcohol. This process involves the use of metal catalysts such as copper or palladium at elevated temperatures to achieve high yields .
Chemical Reactions Analysis
3-Phenyl-2-propenal undergoes various chemical reactions, including:
Oxidation: It can be oxidized to cinnamic acid using oxidizing agents such as potassium permanganate or chromic acid.
Reduction: Reduction of this compound with hydrogen in the presence of a metal catalyst like palladium on carbon yields cinnamyl alcohol.
Substitution: It can undergo nucleophilic addition reactions with compounds like ammonia or amines to form Schiff bases.
Common reagents and conditions used in these reactions include:
Oxidizing agents: Potassium permanganate, chromic acid.
Reducing agents: Hydrogen gas with palladium on carbon.
Nucleophiles: Ammonia, primary amines.
Major products formed from these reactions include cinnamic acid, cinnamyl alcohol, and Schiff bases .
Scientific Research Applications
3-Phenyl-2-propenal has a wide range of applications in scientific research:
Chemistry: It is used as a starting material for the synthesis of various organic compounds, including pharmaceuticals and agrochemicals.
Biology: It exhibits antimicrobial and antifungal properties, making it useful in the development of preservatives and disinfectants.
Medicine: Research has shown that this compound has potential anti-inflammatory and anticancer properties, which are being explored for therapeutic applications.
Mechanism of Action
The mechanism of action of 3-Phenyl-2-propenal involves its interaction with various molecular targets and pathways:
Antimicrobial Activity: It disrupts the cell membrane integrity of microorganisms, leading to cell lysis and death.
Anti-inflammatory Activity: It inhibits the production of pro-inflammatory cytokines and enzymes, thereby reducing inflammation.
Anticancer Activity: It induces apoptosis in cancer cells by activating caspases and other apoptotic pathways.
Comparison with Similar Compounds
3-Phenyl-2-propenal can be compared with other similar compounds such as:
Cinnamic Acid: Unlike this compound, cinnamic acid is a carboxylic acid and is less volatile. It is used in the synthesis of esters and as a precursor for various pharmaceuticals.
Cinnamyl Alcohol: This compound is an alcohol and is used as a fragrance and flavoring agent. It is less reactive compared to this compound.
Cinnamyl Acetate: An ester derivative of cinnamyl alcohol, it is used in perfumes and as a flavoring agent.
This compound stands out due to its unique combination of antimicrobial, anti-inflammatory, and anticancer properties, making it a versatile compound in various fields of research and industry.
Properties
IUPAC Name |
3-phenylprop-2-enal | |
---|---|---|
Details | Computed by Lexichem TK 2.7.0 (PubChem release 2021.05.07) | |
Source | PubChem | |
URL | https://pubchem.ncbi.nlm.nih.gov | |
Description | Data deposited in or computed by PubChem | |
InChI |
InChI=1S/C9H8O/c10-8-4-7-9-5-2-1-3-6-9/h1-8H | |
Details | Computed by InChI 1.0.6 (PubChem release 2021.05.07) | |
Source | PubChem | |
URL | https://pubchem.ncbi.nlm.nih.gov | |
Description | Data deposited in or computed by PubChem | |
InChI Key |
KJPRLNWUNMBNBZ-UHFFFAOYSA-N | |
Details | Computed by InChI 1.0.6 (PubChem release 2021.05.07) | |
Source | PubChem | |
URL | https://pubchem.ncbi.nlm.nih.gov | |
Description | Data deposited in or computed by PubChem | |
Canonical SMILES |
C1=CC=C(C=C1)C=CC=O | |
Details | Computed by OEChem 2.3.0 (PubChem release 2021.05.07) | |
Source | PubChem | |
URL | https://pubchem.ncbi.nlm.nih.gov | |
Description | Data deposited in or computed by PubChem | |
Molecular Formula |
C9H8O | |
Details | Computed by PubChem 2.1 (PubChem release 2021.05.07) | |
Source | PubChem | |
URL | https://pubchem.ncbi.nlm.nih.gov | |
Description | Data deposited in or computed by PubChem | |
DSSTOX Substance ID |
DTXSID1024835 | |
Record name | 3-Phenylprop-2-enal | |
Source | EPA DSSTox | |
URL | https://comptox.epa.gov/dashboard/DTXSID1024835 | |
Description | DSSTox provides a high quality public chemistry resource for supporting improved predictive toxicology. | |
Molecular Weight |
132.16 g/mol | |
Details | Computed by PubChem 2.1 (PubChem release 2021.05.07) | |
Source | PubChem | |
URL | https://pubchem.ncbi.nlm.nih.gov | |
Description | Data deposited in or computed by PubChem | |
CAS No. |
104-55-2 | |
Record name | Cinnamaldehyde | |
Source | CAS Common Chemistry | |
URL | https://commonchemistry.cas.org/detail?cas_rn=104-55-2 | |
Description | CAS Common Chemistry is an open community resource for accessing chemical information. Nearly 500,000 chemical substances from CAS REGISTRY cover areas of community interest, including common and frequently regulated chemicals, and those relevant to high school and undergraduate chemistry classes. This chemical information, curated by our expert scientists, is provided in alignment with our mission as a division of the American Chemical Society. | |
Explanation | The data from CAS Common Chemistry is provided under a CC-BY-NC 4.0 license, unless otherwise stated. | |
Record name | 3-Phenylprop-2-enal | |
Source | EPA DSSTox | |
URL | https://comptox.epa.gov/dashboard/DTXSID1024835 | |
Description | DSSTox provides a high quality public chemistry resource for supporting improved predictive toxicology. | |
Synthesis routes and methods I
Procedure details
Synthesis routes and methods II
Procedure details
Synthesis routes and methods III
Procedure details
Synthesis routes and methods IV
Procedure details
Retrosynthesis Analysis
AI-Powered Synthesis Planning: Our tool employs the Template_relevance Pistachio, Template_relevance Bkms_metabolic, Template_relevance Pistachio_ringbreaker, Template_relevance Reaxys, Template_relevance Reaxys_biocatalysis model, leveraging a vast database of chemical reactions to predict feasible synthetic routes.
One-Step Synthesis Focus: Specifically designed for one-step synthesis, it provides concise and direct routes for your target compounds, streamlining the synthesis process.
Accurate Predictions: Utilizing the extensive PISTACHIO, BKMS_METABOLIC, PISTACHIO_RINGBREAKER, REAXYS, REAXYS_BIOCATALYSIS database, our tool offers high-accuracy predictions, reflecting the latest in chemical research and data.
Strategy Settings
Precursor scoring | Relevance Heuristic |
---|---|
Min. plausibility | 0.01 |
Model | Template_relevance |
Template Set | Pistachio/Bkms_metabolic/Pistachio_ringbreaker/Reaxys/Reaxys_biocatalysis |
Top-N result to add to graph | 6 |
Feasible Synthetic Routes
Disclaimer and Information on In-Vitro Research Products
Please be aware that all articles and product information presented on BenchChem are intended solely for informational purposes. The products available for purchase on BenchChem are specifically designed for in-vitro studies, which are conducted outside of living organisms. In-vitro studies, derived from the Latin term "in glass," involve experiments performed in controlled laboratory settings using cells or tissues. It is important to note that these products are not categorized as medicines or drugs, and they have not received approval from the FDA for the prevention, treatment, or cure of any medical condition, ailment, or disease. We must emphasize that any form of bodily introduction of these products into humans or animals is strictly prohibited by law. It is essential to adhere to these guidelines to ensure compliance with legal and ethical standards in research and experimentation.