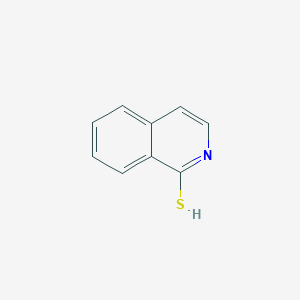
isoquinoline-1-thiol
Overview
Description
Isoquinoline-1-thiol is a heterocyclic aromatic organic compound that contains a sulfur atom attached to the first position of the isoquinoline ring Isoquinoline itself is a structural isomer of quinoline and is composed of a benzene ring fused to a pyridine ring
Preparation Methods
Synthetic Routes and Reaction Conditions
Isoquinoline-1-thiol can be synthesized through several methods. One common approach involves the reaction of isoquinoline with sulfur-containing reagents. For example, isoquinoline can be treated with thiourea in the presence of a strong acid to yield this compound. Another method involves the use of Lawesson’s reagent, which can convert isoquinoline-1-oxide to this compound under mild conditions.
Industrial Production Methods
Industrial production of this compound typically involves the use of large-scale chemical reactors and optimized reaction conditions to ensure high yield and purity. The choice of reagents and catalysts, as well as the control of temperature and pressure, are crucial factors in the industrial synthesis of this compound.
Chemical Reactions Analysis
Types of Reactions
Isoquinoline-1-thiol undergoes various chemical reactions, including:
Oxidation: this compound can be oxidized to form isoquinoline-1-sulfonic acid using oxidizing agents such as hydrogen peroxide or potassium permanganate.
Reduction: Reduction of this compound can yield isoquinoline, especially when using reducing agents like lithium aluminum hydride.
Substitution: this compound can participate in substitution reactions where the thiol group is replaced by other functional groups. For example, reaction with alkyl halides can produce alkylthioisoquinolines.
Common Reagents and Conditions
Oxidation: Hydrogen peroxide, potassium permanganate, and other oxidizing agents.
Reduction: Lithium aluminum hydride, sodium borohydride.
Substitution: Alkyl halides, acyl chlorides, and other electrophiles.
Major Products
Oxidation: Isoquinoline-1-sulfonic acid.
Reduction: Isoquinoline.
Substitution: Alkylthioisoquinolines, acylthioisoquinolines.
Scientific Research Applications
Medicinal Chemistry Applications
Isoquinoline-1-thiol exhibits a range of biological activities, making it a valuable candidate for drug development. Its applications in medicinal chemistry can be categorized as follows:
1.1 Antimicrobial Activity
Research indicates that derivatives of this compound demonstrate significant antimicrobial properties against various bacterial and fungal strains. These compounds have been shown to inhibit the growth of pathogens, thus presenting potential for therapeutic use in treating infections .
1.2 Anticancer Properties
Isoquinoline derivatives, including this compound, have been studied for their anticancer activities. For instance, certain this compound derivatives have been reported to induce apoptosis in cancer cells through mechanisms involving caspase activation . The structure-activity relationship (SAR) studies indicate that modifications to the thiol group can enhance cytotoxicity against specific cancer cell lines .
1.3 Enzyme Inhibition
this compound has been investigated for its potential as an enzyme inhibitor. It can interact with various biological macromolecules, influencing enzymatic pathways critical in disease processes . This makes it a candidate for developing enzyme inhibitors that could lead to new therapeutic agents.
Material Science Applications
The structural characteristics of this compound make it suitable for applications in material science:
2.1 Building Block for Complex Molecules
this compound serves as an essential building block in organic synthesis, facilitating the creation of more complex molecular architectures. Its reactivity due to the thiol group allows for various chemical transformations, which are pivotal in synthesizing novel compounds with desired properties .
2.2 Fluorescent Probes
The unique electronic properties of this compound enable its use as a fluorescent probe in biochemical assays. These probes can be utilized to study cellular processes and interactions at a molecular level, providing insights into biological mechanisms .
Synthetic Approaches and Case Studies
The synthesis of this compound has been explored through various methodologies:
3.1 Synthetic Methodologies
Recent advancements in synthetic techniques have allowed for the efficient construction of this compound and its derivatives. Traditional methods such as the Pictet–Spengler reaction have been adapted to produce these compounds with high yield and selectivity .
3.2 Case Study: Anticancer Activity
A notable study investigated the anticancer effects of this compound derivatives on human cancer cell lines. The results demonstrated that specific modifications to the thiol group significantly enhanced cytotoxicity, leading to increased apoptosis rates in treated cells . This highlights the importance of structural optimization in developing effective anticancer agents.
Comparative Analysis with Related Compounds
To understand the unique features of this compound, a comparative analysis with related compounds is essential:
Compound | Structure Type | Unique Features |
---|---|---|
Cysteine | Amino Acid | Contains a thiol group; crucial for protein structure via disulfide bonds. |
Glutathione | Tripeptide | Acts as a major antioxidant; involved in detoxification processes. |
Mercaptoacetic Acid | Simple Thiol | Used in organic synthesis; less complex than isoquinoline derivatives. |
Thioacetamide | Thioamide | Exhibits different reactivity patterns; used in organic synthesis. |
This compound is distinct due to its combination of a heterocyclic aromatic system and a reactive thiol group, which sets it apart from simpler thiols or other amino acids .
Mechanism of Action
The mechanism of action of isoquinoline-1-thiol involves its interaction with various molecular targets and pathways. The thiol group can form covalent bonds with nucleophilic sites on proteins and enzymes, potentially altering their function. This reactivity can lead to the inhibition of enzyme activity or the modulation of signaling pathways, which may underlie its biological effects.
Comparison with Similar Compounds
Isoquinoline-1-thiol can be compared with other similar compounds such as:
Isoquinoline: Lacks the thiol group and has different chemical reactivity and biological properties.
Quinoline: A structural isomer of isoquinoline with a nitrogen atom at a different position in the ring system.
Thioquinoline: Contains a sulfur atom in the quinoline ring, similar to this compound but with different reactivity and applications.
This compound is unique due to the presence of the thiol group, which imparts distinct chemical and biological properties compared to its analogs.
Q & A
Q. Basic: What are the established synthetic routes for isoquinoline-1-thiol, and how can purity and structural integrity be validated?
Methodological Answer:
this compound is commonly synthesized via transition-metal-free desulfinative cross-coupling of heteroaryl sulfinates. For example, reacting this compound (1.6 g, 10 mmol) under General Procedure A yields a 65% product with characterization via NMR (DO, δ 8.73–7.50) . To ensure purity, combine chromatographic purification (e.g., silica gel) with spectroscopic validation (NMR, IR) and elemental analysis. For novel compounds, X-ray crystallography or high-resolution mass spectrometry (HRMS) is recommended to confirm identity. Cross-referencing with databases like NIST Chemistry WebBook ensures alignment with published spectral data .
Q. Advanced: How does this compound participate in click chemistry reactions, and what experimental controls are critical for optimizing yields?
Methodological Answer:
this compound’s thiol group enables heteroatom-linked click chemistry, such as thiol-ene or thiol-Michael additions. To optimize reactions, control variables like solvent polarity (e.g., DMF vs. water), temperature, and catalyst presence (e.g., base or light for thiol-ene). Monitor reaction progress via TLC or in situ NMR. For reproducibility, include negative controls (e.g., omitting the catalyst) and validate product stability under reaction conditions. Kinetic studies using stopped-flow techniques can elucidate mechanistic pathways, while DFT calculations predict regioselectivity in complex systems .
Q. Basic: What spectroscopic and chromatographic techniques are essential for characterizing this compound derivatives?
Methodological Answer:
Key techniques include:
- NMR : Assign peaks using coupling constants and deuterated solvents (e.g., DO for solubility shifts) .
- HPLC-MS : Quantify purity and detect byproducts; use C18 columns with acetonitrile/water gradients.
- FT-IR : Confirm thiol (-SH) stretches (~2550 cm) and absence of oxidation to disulfides.
For novel derivatives, supplement with NMR, X-ray crystallography, or cyclic voltammetry to assess electronic properties. Tabulate data against reference compounds (e.g., NIST entries) to resolve discrepancies .
Q. Advanced: How can computational methods (e.g., DFT) guide the design of this compound-based catalysts or ligands?
Methodological Answer:
Density Functional Theory (DFT) predicts electronic properties, binding affinities, and reaction pathways. For example:
- Calculate Fukui indices to identify nucleophilic/electrophilic sites for functionalization.
- Simulate transition states to assess activation barriers in catalytic cycles.
- Compare computed NMR chemical shifts (e.g., using Gaussian) with experimental data to validate models.
Integrate computational results with experimental kinetics (e.g., Arrhenius plots) to refine mechanistic hypotheses. Use software like ORCA or NWChem for high-accuracy simulations .
Q. Basic: How should researchers address contradictions in reported physicochemical data (e.g., melting points, NMR shifts) for this compound?
Methodological Answer:
Contradictions may arise from impurities, solvent effects, or instrumentation calibration. To resolve:
Replicate experiments : Reproduce synthesis and characterization under cited conditions.
Cross-validate techniques : Combine DSC (melting point), NMR, and single-crystal XRD for structural confirmation.
Consult authoritative databases : Compare with NIST or peer-reviewed studies for consensus values.
Document all experimental parameters (e.g., heating rates in DSC) to identify variability sources .
Q. Advanced: What strategies optimize this compound’s stability in biological assays, and how are degradation products characterized?
Methodological Answer:
Thiols are prone to oxidation; stabilize via:
- Buffer selection : Use degassed PBS with EDTA to chelate metal catalysts.
- Cryopreservation : Store at -80°C under argon.
Monitor degradation via LC-MS/MS and identify products (e.g., disulfides) using isotopic labeling or tandem mass spectrometry. For in vitro assays, include reducing agents (e.g., TCEP) and validate stability over assay duration via time-course HPLC .
Q. Basic: What are the best practices for documenting synthetic procedures and characterization data in publications?
Methodological Answer:
Follow journal guidelines (e.g., Beilstein Journal of Organic Chemistry):
- Experimental section : Detail stoichiometry, solvents, and purification methods. For known compounds, cite literature; for new compounds, provide full spectroscopic data.
- Supporting information : Include raw NMR/FID files, chromatograms, and crystallographic data (CIF files).
- Reproducibility : Use SI units and specify instrument models (e.g., Bruker 400 MHz NMR) .
Q. Advanced: How can this compound be integrated into metal-organic frameworks (MOFs), and what analytical methods assess host-guest interactions?
Methodological Answer:
Functionalize MOFs via post-synthetic modification (PSM) using thiol-metal coordination. Characterize via:
- PXRD : Confirm framework integrity post-modification.
- BET analysis : Measure surface area changes to confirm grafting.
- XPS : Validate sulfur-metal bonding.
Monitor guest uptake via NMR titration or fluorescence quenching. Computational docking (AutoDock Vina) predicts binding modes .
Q. Basic: What safety protocols are essential when handling this compound in laboratory settings?
Methodological Answer:
- Ventilation : Use fume hoods to avoid inhalation of volatile thiols.
- PPE : Wear nitrile gloves, lab coats, and safety goggles.
- Spill management : Neutralize with oxidizing agents (e.g., NaOCl) and dispose via hazardous waste protocols.
Document risk assessments and Material Safety Data Sheets (MSDS) for institutional compliance .
Q. Advanced: How do solvent effects influence the tautomeric equilibria of this compound, and what spectroscopic methods detect these shifts?
Methodological Answer:
Polar aprotic solvents (e.g., DMSO) stabilize thione tautomers via hydrogen bonding, while nonpolar solvents favor thiol forms. Detect tautomers using:
Properties
IUPAC Name |
isoquinoline-1-thiol | |
---|---|---|
Source | PubChem | |
URL | https://pubchem.ncbi.nlm.nih.gov | |
Description | Data deposited in or computed by PubChem | |
InChI |
InChI=1S/C9H7NS/c11-9-8-4-2-1-3-7(8)5-6-10-9/h1-6H,(H,10,11) | |
Source | PubChem | |
URL | https://pubchem.ncbi.nlm.nih.gov | |
Description | Data deposited in or computed by PubChem | |
InChI Key |
IOYIDSMWPLNHRC-UHFFFAOYSA-N | |
Source | PubChem | |
URL | https://pubchem.ncbi.nlm.nih.gov | |
Description | Data deposited in or computed by PubChem | |
Canonical SMILES |
C1=CC=C2C(=C1)C=CN=C2S | |
Source | PubChem | |
URL | https://pubchem.ncbi.nlm.nih.gov | |
Description | Data deposited in or computed by PubChem | |
Isomeric SMILES |
C1=CC=C2C(=C1)C=CN=C2S | |
Source | PubChem | |
URL | https://pubchem.ncbi.nlm.nih.gov | |
Description | Data deposited in or computed by PubChem | |
Molecular Formula |
C9H7NS | |
Source | PubChem | |
URL | https://pubchem.ncbi.nlm.nih.gov | |
Description | Data deposited in or computed by PubChem | |
Molecular Weight |
161.23 g/mol | |
Source | PubChem | |
URL | https://pubchem.ncbi.nlm.nih.gov | |
Description | Data deposited in or computed by PubChem | |
Disclaimer and Information on In-Vitro Research Products
Please be aware that all articles and product information presented on BenchChem are intended solely for informational purposes. The products available for purchase on BenchChem are specifically designed for in-vitro studies, which are conducted outside of living organisms. In-vitro studies, derived from the Latin term "in glass," involve experiments performed in controlled laboratory settings using cells or tissues. It is important to note that these products are not categorized as medicines or drugs, and they have not received approval from the FDA for the prevention, treatment, or cure of any medical condition, ailment, or disease. We must emphasize that any form of bodily introduction of these products into humans or animals is strictly prohibited by law. It is essential to adhere to these guidelines to ensure compliance with legal and ethical standards in research and experimentation.