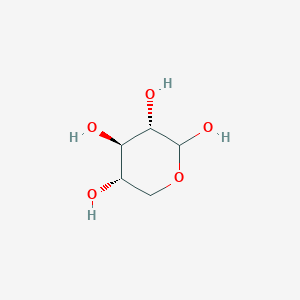
L-Xylopyranose
Overview
Description
L-Xylopyranose is a small molecule that belongs to the class of organic compounds known as pentoses . These are monosaccharides in which the carbohydrate moiety contains five carbon atoms . It is the pyranose form of L-xylose .
Synthesis Analysis
The synthesis of xylopyranosyl donors, as well as protective group chemistry, modifications, and conformational analysis of xylose, has been described in various studies . β-Xylopyranosides have attracted renewed interest due to the development of biomass-derived molecules and their numerous applications .Molecular Structure Analysis
The molecular formula of this compound is C5H10O5 . It has an average mass of 150.130 Da and a monoisotopic mass of 150.052826 Da . It has 3 of 4 defined stereocenters .Chemical Reactions Analysis
The pyrolytic reactivity of β-d-xylopyranose has been thoroughly investigated using computational strategies rooted in quantum chemistry . Its thermal degradation potential energy surfaces have been computed .Physical And Chemical Properties Analysis
This compound is a solid compound . It has a molecular weight of 150.13 g/mol . It has a hydrogen bond donor count of 4 and a hydrogen bond acceptor count of 5 .Scientific Research Applications
Xylooligosaccharides Production and Prebiotic Activity
L-Xylopyranose is a building block of xylooligosaccharides (XOS) obtained from lignocellulosic biomass. XOS demonstrates prebiotic activity and is garnering commercial interest, particularly as a nutraceutical ingredient. The production of XOS involves lignocellulosic biomass pre-treatments, enzymatic hydrolysis of xylan, and further purification through membrane filtration. Despite the variation in methodologies, a two-stage approach involving alkaline pre-treatment and enzymatic hydrolysis is generally recommended. The prebiotic effects of XOS, alongside their production, purification, and characterization, are crucial areas of research (Santibáñez et al., 2021).
Microbial Engineering and Xylonic Acid Management
Mitigating D-xylonic Acid Accumulation in Microorganisms
The xylose oxidative pathway (XOP) is engineered in microorganisms for producing various industrially relevant compounds. D-xylonic acid accumulation, a byproduct, can inhibit microbial growth and enzymatic activity, thus affecting product formation. Strategies including enzyme and evolutionary engineering, coutilization of different sugar substrates, and pathway synergy have been implemented to address this accumulation, which is crucial for improving the efficiency of microbial cell factories (Bañares et al., 2021).
Health Benefits and Applications of Xylitol
Xylitol's Multifaceted Health Benefits
Derived from this compound, xylitol is recognized for its dental health benefits and is explored for various other health advantages. It exhibits a positive impact on skin health, gastrointestinal function, immune modulation, and metabolic health. While it is acknowledged as a low-caloric sweetener contributing to weight management, further research is needed to fully understand its comprehensive benefits and confirm its effectiveness in these areas (Salli et al., 2019).
Xylooligosaccharides: Health Benefits and Food Applications
Functional and Nutritional Importance of Xylooligosaccharides
XOS, derived from this compound, is a potential prebiotic with increasing demand in the food industry. Its functional properties, coupled with its health benefits like improving gut health and immune functions, make it a valuable ingredient in functional foods and nutraceuticals. The physiological importance and biological effects of XOS have been substantiated through various in vitro and in vivo studies, emphasizing its role in the future of food science and technology (Palaniappan et al., 2021).
Safety and Hazards
Future Directions
L-Xylopyranose and its derivatives have potential applications in various industries. For instance, microbial xylanases, which can break down xylan into xylopyranose, are being explored as an “Emerging Green Tool” for various industrial applications . Furthermore, the pyrolytic reactivity of β-d-xylopyranose is being studied for potential applications in biomass conversion .
properties
IUPAC Name |
(3S,4R,5S)-oxane-2,3,4,5-tetrol | |
---|---|---|
Details | Computed by LexiChem 2.6.6 (PubChem release 2019.06.18) | |
Source | PubChem | |
URL | https://pubchem.ncbi.nlm.nih.gov | |
Description | Data deposited in or computed by PubChem | |
InChI |
InChI=1S/C5H10O5/c6-2-1-10-5(9)4(8)3(2)7/h2-9H,1H2/t2-,3+,4-,5?/m0/s1 | |
Details | Computed by InChI 1.0.5 (PubChem release 2019.06.18) | |
Source | PubChem | |
URL | https://pubchem.ncbi.nlm.nih.gov | |
Description | Data deposited in or computed by PubChem | |
InChI Key |
SRBFZHDQGSBBOR-CZBDKTQLSA-N | |
Details | Computed by InChI 1.0.5 (PubChem release 2019.06.18) | |
Source | PubChem | |
URL | https://pubchem.ncbi.nlm.nih.gov | |
Description | Data deposited in or computed by PubChem | |
Canonical SMILES |
C1C(C(C(C(O1)O)O)O)O | |
Details | Computed by OEChem 2.1.5 (PubChem release 2019.06.18) | |
Source | PubChem | |
URL | https://pubchem.ncbi.nlm.nih.gov | |
Description | Data deposited in or computed by PubChem | |
Isomeric SMILES |
C1[C@@H]([C@H]([C@@H](C(O1)O)O)O)O | |
Details | Computed by OEChem 2.1.5 (PubChem release 2019.06.18) | |
Source | PubChem | |
URL | https://pubchem.ncbi.nlm.nih.gov | |
Description | Data deposited in or computed by PubChem | |
Molecular Formula |
C5H10O5 | |
Details | Computed by PubChem 2.1 (PubChem release 2019.06.18) | |
Source | PubChem | |
URL | https://pubchem.ncbi.nlm.nih.gov | |
Description | Data deposited in or computed by PubChem | |
DSSTOX Substance ID |
DTXSID10331418 | |
Record name | L-Xylopyranose | |
Source | EPA DSSTox | |
URL | https://comptox.epa.gov/dashboard/DTXSID10331418 | |
Description | DSSTox provides a high quality public chemistry resource for supporting improved predictive toxicology. | |
Molecular Weight |
150.13 g/mol | |
Details | Computed by PubChem 2.1 (PubChem release 2021.05.07) | |
Source | PubChem | |
URL | https://pubchem.ncbi.nlm.nih.gov | |
Description | Data deposited in or computed by PubChem | |
CAS RN |
19982-83-3 | |
Record name | L-Xylopyranose | |
Source | EPA DSSTox | |
URL | https://comptox.epa.gov/dashboard/DTXSID10331418 | |
Description | DSSTox provides a high quality public chemistry resource for supporting improved predictive toxicology. | |
Retrosynthesis Analysis
AI-Powered Synthesis Planning: Our tool employs the Template_relevance Pistachio, Template_relevance Bkms_metabolic, Template_relevance Pistachio_ringbreaker, Template_relevance Reaxys, Template_relevance Reaxys_biocatalysis model, leveraging a vast database of chemical reactions to predict feasible synthetic routes.
One-Step Synthesis Focus: Specifically designed for one-step synthesis, it provides concise and direct routes for your target compounds, streamlining the synthesis process.
Accurate Predictions: Utilizing the extensive PISTACHIO, BKMS_METABOLIC, PISTACHIO_RINGBREAKER, REAXYS, REAXYS_BIOCATALYSIS database, our tool offers high-accuracy predictions, reflecting the latest in chemical research and data.
Strategy Settings
Precursor scoring | Relevance Heuristic |
---|---|
Min. plausibility | 0.01 |
Model | Template_relevance |
Template Set | Pistachio/Bkms_metabolic/Pistachio_ringbreaker/Reaxys/Reaxys_biocatalysis |
Top-N result to add to graph | 6 |
Feasible Synthetic Routes
Disclaimer and Information on In-Vitro Research Products
Please be aware that all articles and product information presented on BenchChem are intended solely for informational purposes. The products available for purchase on BenchChem are specifically designed for in-vitro studies, which are conducted outside of living organisms. In-vitro studies, derived from the Latin term "in glass," involve experiments performed in controlled laboratory settings using cells or tissues. It is important to note that these products are not categorized as medicines or drugs, and they have not received approval from the FDA for the prevention, treatment, or cure of any medical condition, ailment, or disease. We must emphasize that any form of bodily introduction of these products into humans or animals is strictly prohibited by law. It is essential to adhere to these guidelines to ensure compliance with legal and ethical standards in research and experimentation.