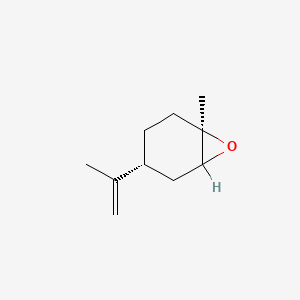
(+)-cis-Limonene 1,2-epoxide
Overview
Description
(+)-cis-Limonene 1,2-epoxide is a useful research compound. Its molecular formula is C10H16O and its molecular weight is 152.23 g/mol. The purity is usually 95%.
BenchChem offers high-quality this compound suitable for many research applications. Different packaging options are available to accommodate customers' requirements. Please inquire for more information about this compound including the price, delivery time, and more detailed information at info@benchchem.com.
Scientific Research Applications
Biocatalysis and Biotransformation
(Carvalho et al., 2000) explored the conversion of cis-limonene-1,2-epoxide to limonene-1,2-diol using Rhodococcus erythropolis DCL14 cells. The study developed an organic/aqueous system to overcome the low solubility and instability of the epoxide. This enabled high production rates of limonene-1,2-diol with a yield of 98.2% for trans-epoxide and 67.9% for the diol.
Asymmetric Synthesis
(Huang et al., 2021) reported on the asymmetric synthesis of 1,2-limonene epoxides. The study achieved controlled absolute stereochemistry through Jacobsen epoxidation, producing cis-1,2-limonene epoxide and trans-1,2-limonene epoxide as raw materials for cannabinoid drugs.
Polymer Synthesis
(Parrino et al., 2018) investigated the reaction of limonene epoxide with carbon dioxide to form polycarbonates with exceptional properties. This study highlights the potential of limonene and carbon dioxide polymers in the bioeconomy.
Enzyme Reactivity and Mechanisms
(Rinaldi et al., 2018) focused on limonene-1,2-epoxide hydrolases (LEHs), exploring their potential for selective hydrolysis of epoxide substrates. The study combined computational methods to understand the structural and dynamic determinants of LEH activity.
Catalysis Research
(Resul et al., 2018) developed a limonene epoxidation process using hydrogen peroxide and a tungsten-based catalyst. This research offers a highly selective and environment-friendly approach for limonene-1,2-epoxide synthesis.
Enzymatic Substrate Specificity
(van der Werf et al., 1999) examined the substrate specificity of limonene-1,2-epoxide hydrolase from Rhodococcus erythropolis DCL14. The study provided insights into the enzyme's narrow substrate range and sequential hydrolysis of limonene-1,2-epoxide diastereomers.
Chemical Synthesis and Purification
(Steiner et al., 2002) explored the kinetic separation of commercially available cis- and trans-limonene epoxide. The study showed that depending on the choice of amine, either cis- or trans-limonene oxide can be obtained in high purity.
Epoxide Hydrolase Research
(Arand et al., 2003) reported the structure of limonene-1,2-epoxide hydrolase from Rhodococcus erythropolis, revealing a novel active site and mechanism. This provides a basis for understanding epoxide hydrolase functions and potential applications.
Epoxide Polymerization
(Soto & Koschek, 2018) studied limonene dioxide polymerization, comparing it with commercial epoxy resins. The research emphasized the influence of diastereoisomeric diversity on the reactivity of epoxy groups in limonene dioxide.
Properties
IUPAC Name |
(1R,4R)-1-methyl-4-prop-1-en-2-yl-7-oxabicyclo[4.1.0]heptane | |
---|---|---|
Details | Computed by Lexichem TK 2.7.0 (PubChem release 2021.05.07) | |
Source | PubChem | |
URL | https://pubchem.ncbi.nlm.nih.gov | |
Description | Data deposited in or computed by PubChem | |
InChI |
InChI=1S/C10H16O/c1-7(2)8-4-5-10(3)9(6-8)11-10/h8-9H,1,4-6H2,2-3H3/t8-,9?,10-/m1/s1 | |
Details | Computed by InChI 1.0.6 (PubChem release 2021.05.07) | |
Source | PubChem | |
URL | https://pubchem.ncbi.nlm.nih.gov | |
Description | Data deposited in or computed by PubChem | |
InChI Key |
CCEFMUBVSUDRLG-RCAUJQPQSA-N | |
Details | Computed by InChI 1.0.6 (PubChem release 2021.05.07) | |
Source | PubChem | |
URL | https://pubchem.ncbi.nlm.nih.gov | |
Description | Data deposited in or computed by PubChem | |
Canonical SMILES |
CC(=C)C1CCC2(C(C1)O2)C | |
Details | Computed by OEChem 2.3.0 (PubChem release 2021.05.07) | |
Source | PubChem | |
URL | https://pubchem.ncbi.nlm.nih.gov | |
Description | Data deposited in or computed by PubChem | |
Isomeric SMILES |
CC(=C)[C@@H]1CC[C@@]2(C(C1)O2)C | |
Details | Computed by OEChem 2.3.0 (PubChem release 2021.05.07) | |
Source | PubChem | |
URL | https://pubchem.ncbi.nlm.nih.gov | |
Description | Data deposited in or computed by PubChem | |
Molecular Formula |
C10H16O | |
Details | Computed by PubChem 2.1 (PubChem release 2021.05.07) | |
Source | PubChem | |
URL | https://pubchem.ncbi.nlm.nih.gov | |
Description | Data deposited in or computed by PubChem | |
Molecular Weight |
152.23 g/mol | |
Details | Computed by PubChem 2.1 (PubChem release 2021.05.07) | |
Source | PubChem | |
URL | https://pubchem.ncbi.nlm.nih.gov | |
Description | Data deposited in or computed by PubChem | |
Retrosynthesis Analysis
AI-Powered Synthesis Planning: Our tool employs the Template_relevance Pistachio, Template_relevance Bkms_metabolic, Template_relevance Pistachio_ringbreaker, Template_relevance Reaxys, Template_relevance Reaxys_biocatalysis model, leveraging a vast database of chemical reactions to predict feasible synthetic routes.
One-Step Synthesis Focus: Specifically designed for one-step synthesis, it provides concise and direct routes for your target compounds, streamlining the synthesis process.
Accurate Predictions: Utilizing the extensive PISTACHIO, BKMS_METABOLIC, PISTACHIO_RINGBREAKER, REAXYS, REAXYS_BIOCATALYSIS database, our tool offers high-accuracy predictions, reflecting the latest in chemical research and data.
Strategy Settings
Precursor scoring | Relevance Heuristic |
---|---|
Min. plausibility | 0.01 |
Model | Template_relevance |
Template Set | Pistachio/Bkms_metabolic/Pistachio_ringbreaker/Reaxys/Reaxys_biocatalysis |
Top-N result to add to graph | 6 |
Feasible Synthetic Routes
Disclaimer and Information on In-Vitro Research Products
Please be aware that all articles and product information presented on BenchChem are intended solely for informational purposes. The products available for purchase on BenchChem are specifically designed for in-vitro studies, which are conducted outside of living organisms. In-vitro studies, derived from the Latin term "in glass," involve experiments performed in controlled laboratory settings using cells or tissues. It is important to note that these products are not categorized as medicines or drugs, and they have not received approval from the FDA for the prevention, treatment, or cure of any medical condition, ailment, or disease. We must emphasize that any form of bodily introduction of these products into humans or animals is strictly prohibited by law. It is essential to adhere to these guidelines to ensure compliance with legal and ethical standards in research and experimentation.