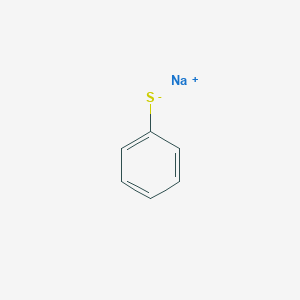
sodium;benzenethiolate
- Click on QUICK INQUIRY to receive a quote from our team of experts.
- With the quality product at a COMPETITIVE price, you can focus more on your research.
Overview
Description
sodium;benzenethiolate, also known as methyltrichlorosilane, is an organosilicon compound with the chemical formula CH₃SiCl₃. It is a colorless liquid with a sharp odor similar to hydrochloric acid. This compound is primarily used as a precursor for forming various cross-linked siloxane polymers .
Preparation Methods
Synthetic Routes and Reaction Conditions: Methyltrichlorosilane is produced through the direct process of reacting chloromethane with elemental silicon in the presence of a copper catalyst at temperatures of at least 250°C . The reaction can be represented as: [ 2 \text{CH}_3\text{Cl} + \text{Si} \rightarrow (\text{CH}_3)_4-n\text{SiCl}_n + \text{other products} ]
Industrial Production Methods: In industrial settings, the production of methyltrichlorosilane involves optimizing the amount of metal catalyst to favor the formation of this compound over other products like dimethyldichlorosilane .
Types of Reactions:
Alcoholysis: Reaction with alcohols to form alkoxysilanes. For example, methanol converts it to trimethoxymethylsilane: [ \text{MeSiCl}_3 + 3 \text{CH}_3\text{OH} \rightarrow \text{MeSi(OCH}_3)_3 + 3 \text{HCl} ]
Reduction: Reduction with alkali metals forms a highly crosslinked material called polymethylsilyne: [ n \text{MeSiCl}_3 + 3n \text{Na} \rightarrow [\text{MeSi}]_n + 3n \text{NaCl} ]
Common Reagents and Conditions:
Hydrolysis: Water
Alcoholysis: Methanol or other alcohols
Reduction: Alkali metals like sodium
Major Products:
Hydrolysis: Methylsilanetriol and hydrochloric acid
Alcoholysis: Trimethoxymethylsilane and hydrochloric acid
Reduction: Polymethylsilyne and sodium chloride
Scientific Research Applications
Methyltrichlorosilane is extensively used in various fields:
Chemistry: As a precursor for synthesizing siloxane polymers and other organosilicon compounds.
Biology: Utilized in the modification of surfaces to create hydrophobic coatings.
Medicine: Employed in the development of biocompatible materials for medical devices.
Industry: Used in the production of silicone resins, adhesives, and sealants.
Mechanism of Action
The primary mechanism by which methyltrichlorosilane exerts its effects is through its reactivity with water and alcohols, leading to the formation of silanols and alkoxysilanes. These intermediates further condense to form cross-linked polymer networks, which are essential in creating various silicone-based materials .
Comparison with Similar Compounds
- Dimethyldichlorosilane (CH₃)₂SiCl₂
- Trimethylchlorosilane (CH₃)₃SiCl
Comparison:
- Dimethyldichlorosilane: Unlike methyltrichlorosilane, it has two methyl groups and two chlorine atoms attached to silicon. It is a major product in the industrial production of organosilicon compounds .
- Trimethylchlorosilane: Contains three methyl groups and one chlorine atom attached to silicon. It is less reactive compared to methyltrichlorosilane due to the presence of more methyl groups .
Methyltrichlorosilane is unique due to its high reactivity and ability to form highly cross-linked polymer networks, making it valuable in various industrial applications .
Properties
IUPAC Name |
sodium;benzenethiolate |
Source
|
---|---|---|
Source | PubChem | |
URL | https://pubchem.ncbi.nlm.nih.gov | |
Description | Data deposited in or computed by PubChem | |
InChI |
InChI=1S/C6H6S.Na/c7-6-4-2-1-3-5-6;/h1-5,7H;/q;+1/p-1 |
Source
|
Source | PubChem | |
URL | https://pubchem.ncbi.nlm.nih.gov | |
Description | Data deposited in or computed by PubChem | |
InChI Key |
RZWQDAUIUBVCDD-UHFFFAOYSA-M |
Source
|
Source | PubChem | |
URL | https://pubchem.ncbi.nlm.nih.gov | |
Description | Data deposited in or computed by PubChem | |
Canonical SMILES |
C1=CC=C(C=C1)[S-].[Na+] |
Source
|
Source | PubChem | |
URL | https://pubchem.ncbi.nlm.nih.gov | |
Description | Data deposited in or computed by PubChem | |
Isomeric SMILES |
C1=CC=C(C=C1)[S-].[Na+] |
Source
|
Source | PubChem | |
URL | https://pubchem.ncbi.nlm.nih.gov | |
Description | Data deposited in or computed by PubChem | |
Molecular Formula |
C6H5NaS |
Source
|
Source | PubChem | |
URL | https://pubchem.ncbi.nlm.nih.gov | |
Description | Data deposited in or computed by PubChem | |
Molecular Weight |
132.16 g/mol |
Source
|
Source | PubChem | |
URL | https://pubchem.ncbi.nlm.nih.gov | |
Description | Data deposited in or computed by PubChem | |
Disclaimer and Information on In-Vitro Research Products
Please be aware that all articles and product information presented on BenchChem are intended solely for informational purposes. The products available for purchase on BenchChem are specifically designed for in-vitro studies, which are conducted outside of living organisms. In-vitro studies, derived from the Latin term "in glass," involve experiments performed in controlled laboratory settings using cells or tissues. It is important to note that these products are not categorized as medicines or drugs, and they have not received approval from the FDA for the prevention, treatment, or cure of any medical condition, ailment, or disease. We must emphasize that any form of bodily introduction of these products into humans or animals is strictly prohibited by law. It is essential to adhere to these guidelines to ensure compliance with legal and ethical standards in research and experimentation.