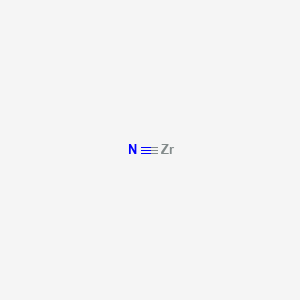
Zirconium nitride
Overview
Description
Zirconium nitride (ZrN) is a transition metal nitride characterized by its exceptional mechanical, thermal, and chemical properties. It crystallizes in a face-centered cubic (FCC) structure and exhibits a golden-yellow hue when deposited as a thin film via physical vapor deposition (PVD) . Key properties include:
- Hardness: 22.7 ± 1.7 GPa (single-crystal) and up to 19.6 GPa for PVD coatings .
- Electrical Resistivity: 12.0 µΩ·cm at room temperature, with a temperature coefficient of 5.6 × 10⁻⁸ Ω·cm/K .
- Thermal Stability: Melting point of ~2,980°C, making it suitable for high-temperature applications .
- Corrosion and Wear Resistance: Excellent resistance to oxidation and chemical degradation, even in aggressive environments .
Preparation Methods
Urea-Based Solvent-Free Synthesis
The urea route represents a low-cost, solvent-free method for synthesizing ZrN fine powders. As demonstrated by Das et al. (2022), ZrCl₄ and urea mixtures are heat-treated at temperatures up to 1600°C under nitrogen gas . Key variables include the ZrCl₄-to-urea molar ratio, heating rate, and dwell time. At a 1:4 molar ratio and 1400°C, single-phase ZrN with a cubic crystal structure (Fm$\overline{3}$m) is obtained, as confirmed by X-ray diffraction (XRD) . Transmission electron microscopy (TEM) reveals particle sizes of 50–100 nm, while energy-dispersive X-ray spectroscopy (EDS) confirms a near-stoichiometric Zr:N ratio of 1:0.98 .
A notable advantage of this method is its avoidance of toxic solvents, reducing environmental impact. However, the requirement for high temperatures (>1400°C) increases energy consumption. Flash sintering of urea-derived ZrN powders achieves near-full density (98.5%) at 80 V/cm and 14 MPa, yielding a Vickers hardness of 15.3 GPa .
Carbothermic Nitridation of Zirconia
Carbothermic nitridation involves reducing zirconia (ZrO₂) with carbon black under nitrogen flow. Ma et al. (2024) optimized this process using internal gel-derived ZrO₂, identifying reaction temperature and carbon-to-zirconium (C/Zr) molar ratio as critical factors . At 1500°C and a C/Zr ratio of 3:1, single-phase ZrN forms via an intermediate zirconium oxynitride (ZrON) phase . Oxygen atoms in ZrON are gradually replaced by nitrogen, facilitated by carbon’s role as an oxygen getter .
Table 1: Phase Evolution in Carbothermic Nitridation
Temperature (°C) | Dwell Time (h) | Phase Composition | Grain Size (nm) |
---|---|---|---|
1200 | 4 | ZrO₂ + ZrON | 25–40 |
1400 | 4 | ZrON + ZrN | 40–60 |
1500 | 2 | ZrN | 80–100 |
This method produces nanopowders with rock-salt crystallography and specific surface areas of 12–18 m²/g . Challenges include residual carbon contamination (0.5–1.2 wt%) and the need for precise stoichiometric control .
Self-Propagating High-Temperature Synthesis (SHS)
SHS leverages exothermic reactions between zirconium metal and nitrogen gas. Zakorzhevskii et al. (2017) achieved 99.5% pure ZrN by igniting Zr powder in a nitrogen atmosphere at 3–5 MPa . The combustion wave propagates at 10–15 mm/s, reaching temperatures exceeding 2500°C, which facilitates rapid nitridation . The resulting powder exhibits a bimodal particle distribution (1–5 µm and 50–100 nm), attributed to partial melting and vapor condensation during cooling .
Advantages:
Limitations:
-
Requires high-purity Zr feedstock (>99.9%) to avoid oxide impurities .
-
Limited control over particle morphology.
Ammonia Nitridation of Metallic Zirconium
A patented method (CN104176716B) utilizes ammonia (NH₃) as the nitrogen source, reacting with 80–120 mesh Zr powder in a rotary kiln at 500–1100°C . NH₃ decomposes into N₂ and H₂, enabling continuous nitridation without pre-reduction. The process yields 99.6% pure ZrN with a cubic structure and particle sizes of 0.5–2 µm .
Key Parameters:
This approach is scalable for industrial production, though NH₃ handling requires stringent safety measures.
Comparative Analysis of Synthesis Methods
Table 2: Method-Specific Outcomes and Trade-offs
Method | Purity (%) | Particle Size | Energy Demand | Scalability |
---|---|---|---|---|
Urea Route | 99.2 | 50–100 nm | High | Lab-scale |
Carbothermic | 98.8 | 80–100 nm | Moderate | Pilot-scale |
SHS | 99.5 | 1–5 µm | Low | Industrial |
Ammonia Nitridation | 99.6 | 0.5–2 µm | Moderate | Industrial |
Scientific Research Applications
Energy Applications
1.1 Fuel Cells
Zirconium nitride has emerged as a promising catalyst in anion exchange membrane fuel cells (AEMFCs). Recent studies have shown that ZrN can outperform platinum in the oxygen reduction reaction (ORR) under alkaline conditions. This capability is attributed to its unique surface properties, which facilitate the adsorption of reactants, thereby enhancing catalytic activity. The study published in Chemical Science outlines a theoretical framework that explains ZrN's superior performance, emphasizing its potential for clean energy applications .
Table 1: Comparison of Catalytic Performance
Catalyst | Activity (mA/cm²) | Cost (USD/g) | Stability |
---|---|---|---|
Platinum | 0.5 | 50 | High |
ZrN | 0.6 | 10 | Moderate |
Fe3N | 0.4 | 5 | Low |
1.2 Nuclear Applications
This compound is also significant in nuclear technology as a ceramic matrix for inert matrix fuels (IMF). Its low neutron capture cross-section and good chemical compatibility with actinides make it suitable for transmuting long-lived isotopes. This application is crucial for developing advanced nuclear fuels that are safer and more efficient .
Biomedical Applications
2.1 Biocompatibility
Research into the biocompatibility of ZrN has revealed its potential for use in bone tissue engineering and protective coatings for implants. Studies have demonstrated that ZrN exhibits no hemolytic activity and shows favorable cytotoxicity profiles, making it a candidate for medical applications .
Table 2: Biocompatibility Properties of ZrN
Property | Result |
---|---|
Hardness | 551.6 gf·mm⁻² |
Density | 6.47 g/cm³ |
Surface Roughness | 0.11 μm |
Cytotoxicity | Non-toxic |
Material Science Applications
3.1 Coatings and Hard Materials
This compound is widely used as a coating material due to its high hardness, thermal stability, and corrosion resistance. It is applied in various industrial settings, including tooling setups for materials processing and as a thermal barrier layer . The high melting point (approximately 2980 °C) allows ZrN to maintain its integrity under extreme conditions.
3.2 Optical Properties
Recent studies have explored the optical properties of ZrN, which are critical for applications in photonics and optoelectronics. The compound exhibits unique dispersion characteristics that can be tailored for specific optical applications .
Case Studies
Case Study 1: Fuel Cell Efficiency Improvement
A recent study demonstrated that integrating ZrN into AEMFCs significantly improved their efficiency compared to traditional catalysts like platinum. The research involved extensive simulations and experimental validations that confirmed ZrN's superior ORR activity under alkaline conditions .
Case Study 2: Biomedical Coating Development
In another study focusing on biomedical applications, researchers synthesized ZrN powders using a gas-solid elemental combination method. The results indicated excellent biocompatibility and mechanical properties suitable for orthopedic implants .
Mechanism of Action
The mechanism by which zirconium nitride exerts its effects is primarily through its physical and chemical properties. Its high hardness and thermal stability make it an effective protective coating, while its electrical conductivity allows it to be used in electronic applications. The molecular targets and pathways involved include the interaction of this compound with various substrates, leading to the formation of strong bonds and enhanced material properties .
Comparison with Similar Compounds
Transition Metal Nitrides
Titanium Nitride (TiN)
- Hardness : ~18–21 GPa, slightly lower than ZrN .
- Electrical Resistivity : 25–70 µΩ·cm, higher than ZrN .
- Applications : Common in decorative coatings and machining tools. ZrN outperforms TiN in high-temperature stability and corrosion resistance .
Tantalum Nitride (TaN)
- Hardness : ~20–23 GPa, comparable to ZrN .
- Thermal Stability : Melting point >3,000°C, superior to ZrN, but less ductile .
- Applications : Used in diffusion barriers for semiconductors. ZrN is preferred for wear-resistant coatings due to lower cost and easier processing .
Table 1: Properties of Transition Metal Nitrides
Other Zirconium Compounds
Zirconium Carbide (ZrC)
- Hardness : ~25 GPa, higher than ZrN, but brittle .
- Thermal Conductivity : ~20 W/m·K, lower than ZrN’s 40–50 W/m·K .
- Applications : Used in nuclear reactors; ZrN is favored for coatings due to better oxidation resistance .
Zirconium Diboride (ZrB₂)
- Hardness : ~23 GPa, similar to ZrN, but with superior thermal shock resistance .
- Electrical Conductivity : Higher than ZrN, making it suitable for electrode materials .
Table 2: Zirconium-Based Compounds
Property | ZrN | ZrC | ZrB₂ |
---|---|---|---|
Hardness (GPa) | 19.6–22.7 | ~25 | ~23 |
Thermal Conductivity | 40–50 | ~20 | 60–70 |
Oxidation Resistance | Excellent | Moderate | Poor |
Key Applications | Coatings | Nuclear | Electrodes |
References |
Ceramic Composites
Silicon Nitride (Si₃N₄)
- Hardness : ~15–18 GPa, lower than ZrN .
- Thermal Conductivity : ~30 W/m·K, comparable to ZrN .
- Applications : Used in bearings and engine components; ZrN excels in environments requiring electrical conductivity .
Aluminum Nitride (AlN)
- Electrical Resistivity : >10¹⁴ Ω·cm, insulating vs. ZrN’s conductive nature .
- Thermal Conductivity : ~170–200 W/m·K, superior to ZrN .
Biocompatible Coatings
Chromium Nitride (CrN)
- Hardness : ~18–20 GPa, slightly lower than ZrN .
- Biocompatibility : ZrN reduces bacterial adhesion by 60% compared to CrN in orthopedic implants .
Table 3: Biocompatible Nitride Coatings
Property | ZrN | CrN | TiN |
---|---|---|---|
Hardness (GPa) | 19.6–22.7 | 18–20 | 18–21 |
Bacterial Adhesion | Low | Moderate | High |
Medical Applications | Orthopedic implants | Cutting tools | Dental implants |
References |
Key Research Findings
- Microstructure Control : ZrN’s grain size and oxygen diffusion can be tailored via pulsed magnetron sputtering, enhancing hardness compared to cathodic arc deposition .
- Doping Effects : Oxygen doping increases ZrN’s tensile strength by 15%, while zirconium vacancies reduce it by 20% .
- Industrial Adoption: ZrN-coated tools exhibit 30% longer lifespan than TiN-coated ones in machining nonferrous metals .
Biological Activity
Zirconium nitride (ZrN) has garnered significant attention in the biomedical field due to its unique properties, including biocompatibility, corrosion resistance, and antimicrobial activity. This article explores the biological activity of ZrN, focusing on its potential applications in biomaterials, particularly in coatings for medical implants and devices.
1. Overview of this compound
This compound is a ceramic compound characterized by its high hardness, thermal stability, and electrical conductivity. These properties make it suitable for various applications, including protective coatings and as a potential biomaterial in medical applications.
2. Biocompatibility
2.1 Cell Adhesion and Proliferation
Recent studies have demonstrated that ZrN coatings promote the adhesion and proliferation of human gingival fibroblasts (HGF). In vitro tests revealed that fibroblasts cultured on ZrN surfaces exhibited significant adhesion rates and proliferative capacity compared to traditional materials like titanium alloys. The MTT assay indicated high cell viability, while immunofluorescence analysis confirmed the production of extracellular matrix components by HGFs on ZrN surfaces .
Table 1: Comparison of Cell Viability on Different Surfaces
Surface Type | Cell Viability (%) | Adhesion Rate (%) |
---|---|---|
Uncoated Ti6Al4V | 70 | 60 |
Anodized Ti | 75 | 65 |
Titanium Nitride | 80 | 70 |
This compound | 90 | 85 |
3. Antimicrobial Properties
3.1 Effectiveness Against Bacterial Strains
This compound has demonstrated significant antimicrobial activity against various bacterial strains, including Staphylococcus aureus and Streptococcus species. In comparative studies, biofilms formed on ZrN surfaces showed a higher percentage of dead bacteria compared to those on titanium nitride surfaces. The presence of silver in ZrN coatings further enhanced its antimicrobial properties, leading to a decrease in viable bacterial counts as silver content increased .
Table 2: Antimicrobial Efficacy of ZrN Coatings
Coating Type | % Live S. aureus | % Live S. epidermidis |
---|---|---|
Ti-ZrN | 2.78 | 0.65 |
Ti-ZrN/6% Ag | 1.30 | 0.52 |
Ti-ZrN/15% Ag | 0.72 | 0.47 |
Ti-ZrN/24% Ag | 0.47 | 0 |
4. Case Studies
4.1 Application in Orthopedic Implants
A study evaluated the performance of ZrN-coated orthopedic implants in a rabbit model. The results indicated that ZrN coatings significantly reduced bacterial colonization on implant surfaces compared to uncoated implants, thereby enhancing the overall success rate of the implants .
4.2 Dental Applications
In dental applications, ZrN coatings have been tested for their ability to inhibit biofilm formation on dental implants. The findings showed that ZrN effectively reduced biofilm density and promoted fibroblast growth, indicating its potential for improving dental implant longevity and success rates .
5. Conclusion
This compound exhibits promising biological activity that supports its application as a biomaterial in various medical fields, particularly in implant technology. Its biocompatibility and antimicrobial properties make it an attractive candidate for enhancing the performance and longevity of medical devices.
6. Future Directions
Further research is needed to explore the long-term effects of ZrN coatings in vivo and to optimize their formulations for specific applications in medicine.
Chemical Reactions Analysis
Oxygen Reduction Reaction (ORR) in Alkaline Media
ZrN exhibits exceptional catalytic activity for ORR in anion exchange membrane fuel cells (AEMFCs), surpassing platinum in alkaline conditions :
Mechanistic Insights
-
Surface State : Under ORR conditions, ZrN surfaces are covered by ~1 monolayer of hydroxyl groups (HO*), which stabilize adsorbates in bridge-site configurations.
-
Electric Field Effects : Minimal dipole moment changes during O* adsorption enable moderate bonding strength, optimizing ORR kinetics .
-
Kinetic Modeling : ZrN operates at the Sabatier optimum in alkaline media, with polarization curves matching experimental data (e.g., 0.9 V vs. RHE at 1 mA/cm²) .
Comparative Performance
Catalyst | Onset Potential (V vs. RHE) | Half-Wave Potential (V vs. RHE) |
---|---|---|
ZrN | 0.95 | 0.85 |
Pt/C | 0.90 | 0.80 |
High-Pressure Phase Transformations
Under high-pressure conditions (6–8 GPa), ZrN forms cubic Zr₃N₄, which decomposes to ZrN at elevated temperatures :
Reaction
Phase Stability
Pressure (GPa) | Temperature (°C) | Stable Phase |
---|---|---|
6.5 | 1400–1600 | c-Zr₃N₄ (+ ls-ZrₓNᵧ) |
7.7 | 1500–1600 | c-Zr₃N₄ |
7.7 | >1800 | ZrN |
Reactivity in Acidic and Oxidizing Environments
ZrN reacts with strong oxidizing agents like chromic acid (CrO₃), producing nitrogen species and metal ions :
Reaction with CrO₃ in H₂SO₄
Products Distribution
Environment | NH₄⁺ Yield (%) | N₂ Yield (%) |
---|---|---|
6M H₂SO₄ + 0.5M CrO₃ | 40–60 | 40–60 |
Hydrogen Permeation and Stability
ZrN films act as hydrogen barriers by forming a composite layer of ZrN and ZrO₂, reducing hydrogen diffusion from zirconium hydride matrices :
Surface Composition (AES Analysis)
Depth (nm) | N (%) | Zr (%) | O (%) |
---|---|---|---|
0–350 | 65 | 30 | 5 |
350–2160 | 50 | 50 | 0 |
-
Hydrogen Interaction : ZrN films reduce hydrogen permeation by 90% compared to uncoated surfaces, attributed to dense microstructure and oxide interlayers .
Reactivity with Other Transition Metal Nitrides
ZrN’s ORR mechanism extends to Fe₃N, TiN, and HfN, suggesting a universal framework for transition metal nitrides in alkaline electrocatalysis .
Q & A
Basic Research Questions
Q. What synthesis methods are commonly used to prepare zirconium nitride (ZrN) thin films, and how do they influence stoichiometry?
- Methodological Answer: ZrN thin films are typically synthesized via physical vapor deposition (PVD) or chemical vapor deposition (CVD). For PVD, reactive magnetron sputtering under controlled nitrogen partial pressure (e.g., 10⁻²–10⁻⁴ mbar) is preferred to optimize stoichiometry. Post-deposition annealing at 800–1000°C in an inert atmosphere can reduce nitrogen vacancies . Characterization via X-ray diffraction (XRD) and energy-dispersive X-ray spectroscopy (EDS) is critical to verify phase purity and N/Zr ratios .
Q. How can nitrogen diffusion coefficients in ZrN be measured experimentally?
- Methodological Answer: Isotopic exchange experiments using ¹⁵N₂ gas coupled with mass spectrometry are standard. Samples (spherical or plate geometries) are heated to 1000–1200°C in a controlled atmosphere, and nitrogen concentration gradients are analyzed over time. Data is fitted to Fick’s second law using computational tools (e.g., finite element analysis) .
Q. What characterization techniques are essential for validating ZrN’s structural and thermal stability?
- Methodological Answer: High-resolution transmission electron microscopy (HRTEM) and X-ray photoelectron spectroscopy (XPS) are used to assess crystallinity and surface oxidation resistance. Thermogravimetric analysis (TGA) under varying oxygen partial pressures quantifies thermal stability up to 1200°C .
Advanced Research Questions
Q. How do sample geometry and vacancy concentrations affect discrepancies in reported nitrogen diffusion coefficients for ZrN?
- Methodological Answer: Spherical samples exhibit lower diffusion coefficients (D ≈ 2.97×10⁻¹⁰ exp(-23,000/RT) cm²/s) compared to plates (D ≈ 100× higher) due to differences in vacancy distribution and surface-to-volume ratios. To resolve contradictions, future studies should systematically vary sample mass, chamber pressure, and geometry while using probabilistic models to account for vacancy-driven diffusion anomalies .
Q. What statistical frameworks are recommended to interpret conflicting data in ZrN diffusion studies?
- Methodological Answer: Use 95% confidence intervals (CI) and least-squares regression to assess uncertainty in Arrhenius parameters (e.g., pre-exponential factor D₀ and activation energy Q). For non-linear diffusion trends (e.g., time-dependent D decay), segmented regression or Bayesian hierarchical modeling can separate surface reaction-controlled vs. bulk diffusion mechanisms .
Q. How can multidisciplinary approaches (e.g., electrochemistry and solid-state kinetics) enhance understanding of ZrN’s reactivity?
- Methodological Answer: Integrate electrochemical impedance spectroscopy (EIS) with high-temperature XRD to correlate ionic conductivity with phase transitions. Reference prior work on ZrN’s electronic structure (e.g., density functional theory studies) to predict defect-mediated diffusion pathways. Cross-validation with in situ Raman spectroscopy during thermal cycling is advised .
Q. Data Interpretation & Contradictions
Q. Why do nitrogen diffusion rates in ZrN deviate from Arrhenius behavior at temperatures above 1100°C?
- Methodological Answer: Deviations arise due to competing mechanisms like grain boundary diffusion and vacancy clustering. At >1100°C, rapid surface reactions dominate, invalidating bulk diffusion assumptions. Use time-resolved mass spectrometry and Monte Carlo simulations to decouple these contributions .
Q. How should researchers address inconsistencies in literature-reported activation energies for ZrN oxidation?
- Methodological Answer: Perform meta-analyses of existing studies, controlling for variables like impurity levels (e.g., oxygen content) and measurement techniques. Experimental replication using standardized TGA protocols (e.g., 5°C/min heating rate in dry air) is critical. Report uncertainties using expanded error margins (±2σ) .
Q. Methodological Frameworks
Q. What frameworks (e.g., PICO, FINER) are suitable for structuring ZrN research questions?
- Methodological Answer: Apply the FINER criteria (Feasible, Interesting, Novel, Ethical, Relevant) to prioritize studies on ZrN’s high-temperature stability. For clinical or applied studies, use PICO (Population: ZrN coatings; Intervention: doping with Al/Ti; Comparison: undoped ZrN; Outcome: corrosion resistance) .
Q. How can probabilistic models improve the design of ZrN diffusion experiments?
- Methodological Answer: Bayesian inference models incorporating prior data (e.g., from isotope exchange experiments) reduce sample size requirements. Use Markov chain Monte Carlo (MCMC) sampling to optimize parameters like gas pressure (S/N ratio) and temperature gradients, minimizing experimental iterations .
Q. Tables of Key Data
Table 1. Nitrogen Diffusion Coefficients in ZrN (Selected Studies)
Temperature (°C) | Geometry | D (cm²/s) | Method | Reference |
---|---|---|---|---|
1053 | Sphere | 3.2×10⁻¹¹ | Isotopic exchange | |
1101 | Plate | 1.1×10⁻⁹ | Mass spectrometry | |
1203 | Sphere | 8.7×10⁻¹¹ | Finite element analysis |
Table 2. Common Pitfalls in ZrN Research Design
Pitfall | Mitigation Strategy |
---|---|
Ignoring sample geometry effects | Use standardized geometries (e.g., ASTM B214 for powders) |
Overlooking gas purity | Employ gas chromatographs to monitor N₂/O₂ ratios |
Inadequate statistical power | Precalculate sample sizes using G*Power or similar tools |
Properties
IUPAC Name |
azanylidynezirconium | |
---|---|---|
Source | PubChem | |
URL | https://pubchem.ncbi.nlm.nih.gov | |
Description | Data deposited in or computed by PubChem | |
InChI |
InChI=1S/N.Zr | |
Source | PubChem | |
URL | https://pubchem.ncbi.nlm.nih.gov | |
Description | Data deposited in or computed by PubChem | |
InChI Key |
ZVWKZXLXHLZXLS-UHFFFAOYSA-N | |
Source | PubChem | |
URL | https://pubchem.ncbi.nlm.nih.gov | |
Description | Data deposited in or computed by PubChem | |
Canonical SMILES |
N#[Zr] | |
Source | PubChem | |
URL | https://pubchem.ncbi.nlm.nih.gov | |
Description | Data deposited in or computed by PubChem | |
Molecular Formula |
ZrN, NZr | |
Record name | zirconium nitride | |
Source | Wikipedia | |
URL | https://en.wikipedia.org/wiki/Zirconium_nitride | |
Description | Chemical information link to Wikipedia. | |
Source | PubChem | |
URL | https://pubchem.ncbi.nlm.nih.gov | |
Description | Data deposited in or computed by PubChem | |
DSSTOX Substance ID |
DTXSID2067119 | |
Record name | Zirconium nitride (ZrN) | |
Source | EPA DSSTox | |
URL | https://comptox.epa.gov/dashboard/DTXSID2067119 | |
Description | DSSTox provides a high quality public chemistry resource for supporting improved predictive toxicology. | |
Molecular Weight |
105.23 g/mol | |
Source | PubChem | |
URL | https://pubchem.ncbi.nlm.nih.gov | |
Description | Data deposited in or computed by PubChem | |
Physical Description |
Yellow to brown odorless powder; [Alfa Aesar MSDS] Insoluble in water; [Wikipedia] | |
Record name | Zirconium nitride | |
Source | Haz-Map, Information on Hazardous Chemicals and Occupational Diseases | |
URL | https://haz-map.com/Agents/18750 | |
Description | Haz-Map® is an occupational health database designed for health and safety professionals and for consumers seeking information about the adverse effects of workplace exposures to chemical and biological agents. | |
Explanation | Copyright (c) 2022 Haz-Map(R). All rights reserved. Unless otherwise indicated, all materials from Haz-Map are copyrighted by Haz-Map(R). No part of these materials, either text or image may be used for any purpose other than for personal use. Therefore, reproduction, modification, storage in a retrieval system or retransmission, in any form or by any means, electronic, mechanical or otherwise, for reasons other than personal use, is strictly prohibited without prior written permission. | |
CAS No. |
25658-42-8, 12033-93-1 | |
Record name | Zirconium nitride (ZrN) | |
Source | CAS Common Chemistry | |
URL | https://commonchemistry.cas.org/detail?cas_rn=25658-42-8 | |
Description | CAS Common Chemistry is an open community resource for accessing chemical information. Nearly 500,000 chemical substances from CAS REGISTRY cover areas of community interest, including common and frequently regulated chemicals, and those relevant to high school and undergraduate chemistry classes. This chemical information, curated by our expert scientists, is provided in alignment with our mission as a division of the American Chemical Society. | |
Explanation | The data from CAS Common Chemistry is provided under a CC-BY-NC 4.0 license, unless otherwise stated. | |
Record name | Zirconium nitride (Zr3N4) | |
Source | CAS Common Chemistry | |
URL | https://commonchemistry.cas.org/detail?cas_rn=12033-93-1 | |
Description | CAS Common Chemistry is an open community resource for accessing chemical information. Nearly 500,000 chemical substances from CAS REGISTRY cover areas of community interest, including common and frequently regulated chemicals, and those relevant to high school and undergraduate chemistry classes. This chemical information, curated by our expert scientists, is provided in alignment with our mission as a division of the American Chemical Society. | |
Explanation | The data from CAS Common Chemistry is provided under a CC-BY-NC 4.0 license, unless otherwise stated. | |
Record name | Zirconium nitride (ZrN) | |
Source | ChemIDplus | |
URL | https://pubchem.ncbi.nlm.nih.gov/substance/?source=chemidplus&sourceid=0025658428 | |
Description | ChemIDplus is a free, web search system that provides access to the structure and nomenclature authority files used for the identification of chemical substances cited in National Library of Medicine (NLM) databases, including the TOXNET system. | |
Record name | Zirconium nitride (ZrN) | |
Source | EPA Chemicals under the TSCA | |
URL | https://www.epa.gov/chemicals-under-tsca | |
Description | EPA Chemicals under the Toxic Substances Control Act (TSCA) collection contains information on chemicals and their regulations under TSCA, including non-confidential content from the TSCA Chemical Substance Inventory and Chemical Data Reporting. | |
Record name | Zirconium nitride (ZrN) | |
Source | EPA DSSTox | |
URL | https://comptox.epa.gov/dashboard/DTXSID2067119 | |
Description | DSSTox provides a high quality public chemistry resource for supporting improved predictive toxicology. | |
Record name | Zirconium nitride | |
Source | European Chemicals Agency (ECHA) | |
URL | https://echa.europa.eu/substance-information/-/substanceinfo/100.042.864 | |
Description | The European Chemicals Agency (ECHA) is an agency of the European Union which is the driving force among regulatory authorities in implementing the EU's groundbreaking chemicals legislation for the benefit of human health and the environment as well as for innovation and competitiveness. | |
Explanation | Use of the information, documents and data from the ECHA website is subject to the terms and conditions of this Legal Notice, and subject to other binding limitations provided for under applicable law, the information, documents and data made available on the ECHA website may be reproduced, distributed and/or used, totally or in part, for non-commercial purposes provided that ECHA is acknowledged as the source: "Source: European Chemicals Agency, http://echa.europa.eu/". Such acknowledgement must be included in each copy of the material. ECHA permits and encourages organisations and individuals to create links to the ECHA website under the following cumulative conditions: Links can only be made to webpages that provide a link to the Legal Notice page. | |
Disclaimer and Information on In-Vitro Research Products
Please be aware that all articles and product information presented on BenchChem are intended solely for informational purposes. The products available for purchase on BenchChem are specifically designed for in-vitro studies, which are conducted outside of living organisms. In-vitro studies, derived from the Latin term "in glass," involve experiments performed in controlled laboratory settings using cells or tissues. It is important to note that these products are not categorized as medicines or drugs, and they have not received approval from the FDA for the prevention, treatment, or cure of any medical condition, ailment, or disease. We must emphasize that any form of bodily introduction of these products into humans or animals is strictly prohibited by law. It is essential to adhere to these guidelines to ensure compliance with legal and ethical standards in research and experimentation.