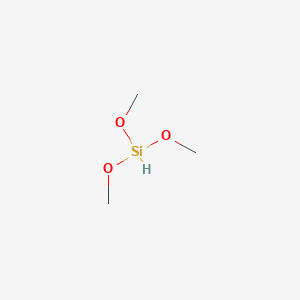
trimethoxysilane
Overview
Description
Trimethoxysilane derivatives are organosilicon compounds characterized by a central silicon atom bonded to three methoxy (–OCH₃) groups and one organofunctional group. These compounds serve as critical coupling agents in materials science, enabling interfacial bonding between inorganic substrates (e.g., metals, glass, or nanoparticles) and organic polymers. The methoxy groups hydrolyze in the presence of moisture or catalysts, forming silanol (–SiOH) intermediates that condense to create covalent bonds with hydroxylated surfaces. The organofunctional group (e.g., amino, mercapto, or epoxy) determines the compound’s reactivity and compatibility with polymers, facilitating applications in composites, coatings, adhesives, and biomedical devices .
For example, (3-aminopropyl)this compound (APTS) is widely used to functionalize carbon nanofibers (CNF) or magnetite nanoparticles, enhancing dispersion in polymer matrices and enabling covalent bonding with epoxy resins or biomolecules . Similarly, (3-mercaptopropyl)this compound (MPTS) introduces thiol groups for gold nanoparticle conjugation in biosensors and photothermal materials .
Preparation Methods
Trimethoxysilane can be synthesized through several methods. One common method involves the direct synthesis of trialkoxysilane by reacting silicon with methanol in the presence of a copper catalyst . This reaction can be carried out in a solvent with a catalytically effective amount of a direct synthesis catalyst and a catalyst promoter, often an organic or inorganic compound possessing at least one phosphorus-oxygen bond . Another method involves using a wet chemical reduction method to prepare nano-copper, followed by preparing a silicon powder-nano-copper catalyst mixture, and then producing this compound in a fixed bed reactor .
Chemical Reactions Analysis
Hydrolysis Reactions
Trimethoxysilane undergoes rapid hydrolysis in aqueous environments, forming methanol and silanetriol (HSi(OH)₃) as primary products .
Kinetics and Mechanisms
-
Rate and Half-Life :
Hydrolysis occurs within seconds to minutes, depending on conditions: -
pH Dependence :
Condition | Mechanism | Rate Constant (k) | Activation Energy (Eₐ) | Source |
---|---|---|---|---|
Acidic (HCl) | SN1-Si | 5.5–97 mM⁻¹ h⁻¹* | 28.1 kJ/mol† | |
Alkaline (NH₄OH) | SN2-Si | 2.453 × 10⁴ sec⁻¹‡ | 50.09 kJ/mol‡ |
*Varies with substituents (e.g., mercaptopropyl vs. methyl groups) .
†For mercaptopropyl this compound (MPTMS) .
‡For methylthis compound (MTMS) .
Condensation Reactions
Silanetriol (HSi(OH)₃) undergoes condensation to form siloxane polymers (Si-O-Si networks) .
Kinetics and Catalysis
-
Base-Catalyzed Condensation :
-
Acid-Catalyzed Condensation :
Produces less branched structures due to preferential reaction with less acidic silanol groups .
Parameter | Value (MPTMS) | Value (MTMS) | Source |
---|---|---|---|
Reaction Order (Silanol) | 1.4 | Not reported | |
Reaction Order (Catalyst) | 2.8 | Not reported | |
Pre-exponential Factor (A) | 7.5×10¹³ (mol/L)⁻³·²·min⁻¹ | 2.453 × 10⁴ sec⁻¹ |
Process Details
Reactor Parameter | Optimal Value | Impact on Yield | Source |
---|---|---|---|
Number of Reactors | 3–4 (serial setup) | Increases methanol conversion | |
Methanol Feed Rate | Countercurrent flow | Reduces azeotrope formation |
Environmental Degradation
This compound rapidly hydrolyzes in the atmosphere, with secondary degradation via hydroxyl radicals .
Degradation Pathways
-
Hydrolysis : Dominates in humid air, yielding methanol and silanetriol .
-
Photolysis : Minimal contribution; half-life of 6.4 days via OH radical attack .
-
Silanetriol Oxidation : Further reacts with OH radicals (half-life: 1.6 days) .
Degradation Route | Half-Life | Products | Source |
---|---|---|---|
Hydrolysis (air) | 3 minutes | Methanol, silanetriol | |
OH Radical Attack | 6.4 days | CO₂, H₂O |
Key Reaction Example
Synthesis of Trimethoxysilylpropyl Methacrylate :
Scientific Research Applications
Applications in Materials Science
- Coupling Agent : Trimethoxysilane is widely used as a coupling agent in the formulation of composite materials. It improves the interfacial adhesion between organic polymers and inorganic fillers, enhancing mechanical properties such as tensile strength and impact resistance .
- Surface Modification : This compound can modify the surface properties of materials, including wettability and hydrophobicity. For instance, it can enhance the water resistance of coatings and improve the performance of composites by creating a hydrophobic layer .
Coatings and Sealants
- Protective Coatings : this compound is employed in protective coatings for metals, enhancing corrosion resistance and durability. It forms a siloxane network that provides a barrier against environmental factors .
- Adhesion Promoter : In silicone sealants and adhesives, this compound acts as an adhesion promoter, improving the bond strength between substrates and sealant materials .
Case Study 1: Composite Materials
A study demonstrated that incorporating this compound into epoxy resin composites significantly improved their mechanical properties. The treated composites exhibited enhanced tensile strength and impact resistance compared to untreated samples .
Case Study 2: Coating Formulations
Research on automotive coatings revealed that adding this compound improved the adhesion of paint to metal surfaces, resulting in longer-lasting finishes that resist chipping and peeling under harsh conditions .
Applications in Electronics
- Electrode Fabrication : this compound has been utilized in the fabrication of vapor-deposited gold electrodes on glass substrates. Its ability to form stable bonds enhances the conductivity and overall performance of electronic devices .
Environmental Applications
Mechanism of Action
The mechanism of action of trimethoxysilane involves its hydrolysis to form silanol groups, which can then condense to form siloxane bonds . This process allows it to act as a coupling agent, forming strong bonds between different materials . The molecular targets and pathways involved include the interaction of silanol groups with hydroxyl groups on surfaces, leading to the formation of covalent bonds .
Comparison with Similar Compounds
Comparison with Similar Trimethoxysilane Derivatives
Functional Groups and Reactivity
The organofunctional group dictates the chemical reactivity and application scope of trimethoxysilanes:
Key Findings :
- APTS outperforms (3-aminopropyl)triethoxysilane (APTES) in hydrolysis rate due to smaller methoxy groups, enabling faster surface bonding .
- MPTS-grafted surfaces exhibit superior stability in aqueous environments compared to amino-functionalized silanes, critical for biosensor durability .
Physical and Surface Properties
Alkyl chain length and fluorination significantly influence hydrophobicity and optical properties:
Table 2: Wettability of Alkyl Trimethoxysilanes (0.09 M Precursor)
Compound | Water Contact Angle (°) | Sliding Angle (°) | Transmittance (%) |
---|---|---|---|
Vinyl this compound (VTMS) | 75 | 28 | 89 |
Octadecyl this compound | 112 | 8 | 83 |
Propyl this compound | 68 | 35 | 91 |
Decyl this compound | 98 | 15 | 86 |
Fluorinated derivatives (e.g., FAS17) achieve ultrahigh hydrophobicity (contact angle >150°) but reduce optical clarity, making them ideal for anti-fouling coatings but unsuitable for transparent films .
Performance in Polymer Composites
Silane treatment enhances mechanical and electrical properties of acrylonitrile-butadiene rubber (NBR) composites:
Table 3: Mechanical Properties of NBR/CNF Composites
Silane Treatment | Tensile Strength (MPa) | Elongation at Break (%) | Electrical Conductivity (S/m) |
---|---|---|---|
Untreated CNF | 8.2 | 320 | 0.03 |
APTS (chemical silanization) | 14.7 | 290 | 0.45 |
MPTS (in-situ silanization) | 12.1 | 310 | 0.28 |
UPTS (chemical silanization) | 13.9 | 280 | 0.39 |
Key Findings :
Biological Activity
Trimethoxysilane (TMS) is a silane compound that has garnered attention for its various biological activities, particularly in antimicrobial applications and its interactions with biological systems. This article explores the biological activity of this compound, supported by research findings, case studies, and data tables.
Overview of this compound
This compound is a silane compound characterized by the presence of three methoxy groups attached to a silicon atom. It is commonly used in surface modification, as a coupling agent in composites, and as a precursor in the synthesis of silicate materials. Its hydrolytic instability makes it reactive in aqueous environments, leading to the formation of silanol groups that can interact with various substrates.
Antimicrobial Activity
Mechanism of Action
This compound exhibits significant antibacterial properties, particularly when modified or combined with other compounds. For instance, a study synthesized (3-trimethoxysilylpropyl)quinolinium iodide (TMSQI), which demonstrated strong antibacterial activity against both Gram-positive and Gram-negative bacteria. The mechanism proposed involves electrostatic interactions between the positively charged TMSQI and the negatively charged bacterial cell membranes, leading to membrane disruption and cell death .
Case Study: Antibacterial Efficacy
In a study involving nanofibrillated cellulose (NFC) modified with TMSQI, researchers observed a notable reduction in bacterial concentration. The zones of inhibition ranged from 10 mm to 20 mm against various bacterial strains. The effectiveness was influenced by the concentration of the silane compound used during grafting .
Bacterial Strain | Zone of Inhibition (mm) |
---|---|
Staphylococcus aureus | 15 |
Escherichia coli | 12 |
Pseudomonas aeruginosa | 18 |
Toxicological Studies
Inhalation Toxicity
Research on the inhalation toxicity of this compound indicates that it primarily affects the respiratory system. In rat studies, exposure to concentrations as low as 5 ppm resulted in lethality due to respiratory tract injury. The No Observed Adverse Effect Concentration (NOAEC) was determined to be around 0.5 ppm, suggesting that while TMS can be toxic at higher concentrations, its effects are localized primarily to the respiratory system without systemic toxicity .
Genotoxicity Assessment
This compound has been evaluated for genotoxic potential through various assays. It was found to be non-mutagenic in vitro in bacterial reverse mutation tests and did not induce micronuclei formation in vivo. These findings suggest that this compound is unlikely to pose significant genotoxic risks under typical exposure scenarios .
Applications in Biomedical Fields
Surface Functionalization
This compound is extensively used for surface functionalization in biomedical applications. Its ability to form self-assembled monolayers allows for enhanced adhesion properties on various substrates, which is crucial for medical devices and tissue engineering applications .
Q & A
Basic Research Questions
Q. What are the established methods for synthesizing trimethoxysilane derivatives, and how do reaction conditions influence yield?
- This compound derivatives, such as (3-mercaptopropyl)this compound (MPTMS) or (3-aminopropyl)this compound (APTMS), are typically synthesized via copper-catalyzed reactions. For example, Suzuki and Ono (1990) demonstrated that copper catalysts facilitate the reaction between silicon and methanol to produce this compound under controlled temperature (150–200°C) and pressure (1–3 atm) . Key factors affecting yield include:
- Catalyst concentration : Excess copper may lead to side reactions.
- Solvent choice : Polar aprotic solvents (e.g., THF) improve silane stability.
- Moisture control : Hydrolysis of methoxy groups must be minimized to avoid premature crosslinking .
Q. What safety protocols are critical when handling this compound in laboratory settings?
- This compound is highly flammable (flash point: 23°C) and poses severe inhalation and dermal hazards. Mandatory precautions include:
- Ventilation : Use fume hoods for all procedures involving liquid or vapor phases.
- PPE : Nitrile gloves, chemical goggles, and flame-resistant lab coats.
- Emergency response : Immediate rinsing with water for skin/eye contact (15+ minutes) and medical consultation .
- Acute Exposure Guideline Levels (AEGLs) for this compound are not formally established, but rodent studies indicate LC₅₀ values < 500 ppm for inhalation .
Q. How can researchers determine the purity and structural integrity of this compound derivatives?
- Key analytical methods:
- Gas chromatography (GC) : Quantifies residual methanol or byproducts.
- FTIR spectroscopy : Identifies functional groups (e.g., Si-O-CH₃ at 1080 cm⁻¹, SH stretch at 2550 cm⁻¹ for MPTMS) .
- NMR : ¹H and ²⁹Si NMR confirm methoxy group integrity and silane condensation .
Advanced Research Questions
Q. What advanced separation techniques are effective for isolating this compound-methanol azeotropes?
- The this compound/methanol azeotrope (boiling point: 64°C at 1 atm) can be separated via pressure-swing distillation (PSD). Luyben (2014) optimized a two-column PSD system:
- First column : Operates at 0.5 atm to break the azeotrope, yielding 99% pure methanol.
- Second column : Operates at 4 atm to recover this compound with >98% purity .
Q. How does surface functionalization with this compound enhance material performance in nanocomposites?
- Trimethoxysilanes act as coupling agents to improve interfacial adhesion in composites. For example:
- Enzyme immobilization : APTMS-functionalized nanozeolites increase lipase loading by 40% via glutaraldehyde crosslinking, enhancing catalytic activity in biodiesel production .
- Dental materials : Silane grafting (e.g., MPTMS) on PMMA improves bond strength to soft liners by 300% due to siloxane (Si-O-Si) crosslinking, confirmed via XPS and FTIR .
Q. How can researchers resolve contradictions in catalytic efficiency reports for silane-mediated reactions?
- Discrepancies in catalytic performance (e.g., APTMS vs. GLYMO in nanoparticle functionalization) arise from:
- Surface heterogeneity : APTMS-modified iron oxide agglomerates more readily than GLYMO-functionalized particles, reducing active sites .
- Hydrolysis kinetics : APTMS hydrolyzes faster in aqueous media, leading to premature condensation and reduced stability .
- Mitigation strategies :
- Use non-aqueous solvents (e.g., toluene) for APTMS grafting.
- Optimize pH (5–6) to balance hydrolysis and condensation rates .
Q. What methodologies enable precise analysis of silane grafting efficiency on solid substrates?
- Quantitative FTIR : Peak height ratios (e.g., Si-O-CH₃/Si-O-Si) correlate with grafting density .
- X-ray photoelectron spectroscopy (XPS) : Measures atomic % of silicon and oxygen to confirm monolayer formation. For APTMS on steel, a Si atomic % >2.5 indicates successful grafting .
- Contact angle analysis : Wettability changes (e.g., 30° reduction for APTMS-grafted galvannealed steel) validate surface modification .
Properties
IUPAC Name |
trimethoxysilane | |
---|---|---|
Details | Computed by LexiChem 2.6.6 (PubChem release 2019.06.18) | |
Source | PubChem | |
URL | https://pubchem.ncbi.nlm.nih.gov | |
Description | Data deposited in or computed by PubChem | |
InChI |
InChI=1S/C3H10O3Si/c1-4-7(5-2)6-3/h7H,1-3H3 | |
Details | Computed by InChI 1.0.5 (PubChem release 2019.06.18) | |
Source | PubChem | |
URL | https://pubchem.ncbi.nlm.nih.gov | |
Description | Data deposited in or computed by PubChem | |
InChI Key |
YUYCVXFAYWRXLS-UHFFFAOYSA-N | |
Details | Computed by InChI 1.0.5 (PubChem release 2019.06.18) | |
Source | PubChem | |
URL | https://pubchem.ncbi.nlm.nih.gov | |
Description | Data deposited in or computed by PubChem | |
Canonical SMILES |
CO[SiH](OC)OC | |
Details | Computed by OEChem 2.1.5 (PubChem release 2019.06.18) | |
Source | PubChem | |
URL | https://pubchem.ncbi.nlm.nih.gov | |
Description | Data deposited in or computed by PubChem | |
Molecular Formula |
C3H10O3Si | |
Details | Computed by PubChem 2.1 (PubChem release 2019.06.18) | |
Source | PubChem | |
URL | https://pubchem.ncbi.nlm.nih.gov | |
Description | Data deposited in or computed by PubChem | |
Related CAS |
153233-53-5 | |
Details | Compound: Silane, trimethoxy-, homopolymer | |
Record name | Silane, trimethoxy-, homopolymer | |
Source | CAS Common Chemistry | |
URL | https://commonchemistry.cas.org/detail?cas_rn=153233-53-5 | |
Description | CAS Common Chemistry is an open community resource for accessing chemical information. Nearly 500,000 chemical substances from CAS REGISTRY cover areas of community interest, including common and frequently regulated chemicals, and those relevant to high school and undergraduate chemistry classes. This chemical information, curated by our expert scientists, is provided in alignment with our mission as a division of the American Chemical Society. | |
Explanation | The data from CAS Common Chemistry is provided under a CC-BY-NC 4.0 license, unless otherwise stated. | |
Molecular Weight |
122.19 g/mol | |
Details | Computed by PubChem 2.1 (PubChem release 2021.05.07) | |
Source | PubChem | |
URL | https://pubchem.ncbi.nlm.nih.gov | |
Description | Data deposited in or computed by PubChem | |
CAS No. |
2487-90-3 | |
Record name | Trimethoxysilane | |
Source | CAS Common Chemistry | |
URL | https://commonchemistry.cas.org/detail?cas_rn=2487-90-3 | |
Description | CAS Common Chemistry is an open community resource for accessing chemical information. Nearly 500,000 chemical substances from CAS REGISTRY cover areas of community interest, including common and frequently regulated chemicals, and those relevant to high school and undergraduate chemistry classes. This chemical information, curated by our expert scientists, is provided in alignment with our mission as a division of the American Chemical Society. | |
Explanation | The data from CAS Common Chemistry is provided under a CC-BY-NC 4.0 license, unless otherwise stated. | |
Synthesis routes and methods
Procedure details
Retrosynthesis Analysis
AI-Powered Synthesis Planning: Our tool employs the Template_relevance Pistachio, Template_relevance Bkms_metabolic, Template_relevance Pistachio_ringbreaker, Template_relevance Reaxys, Template_relevance Reaxys_biocatalysis model, leveraging a vast database of chemical reactions to predict feasible synthetic routes.
One-Step Synthesis Focus: Specifically designed for one-step synthesis, it provides concise and direct routes for your target compounds, streamlining the synthesis process.
Accurate Predictions: Utilizing the extensive PISTACHIO, BKMS_METABOLIC, PISTACHIO_RINGBREAKER, REAXYS, REAXYS_BIOCATALYSIS database, our tool offers high-accuracy predictions, reflecting the latest in chemical research and data.
Strategy Settings
Precursor scoring | Relevance Heuristic |
---|---|
Min. plausibility | 0.01 |
Model | Template_relevance |
Template Set | Pistachio/Bkms_metabolic/Pistachio_ringbreaker/Reaxys/Reaxys_biocatalysis |
Top-N result to add to graph | 6 |
Feasible Synthetic Routes
Disclaimer and Information on In-Vitro Research Products
Please be aware that all articles and product information presented on BenchChem are intended solely for informational purposes. The products available for purchase on BenchChem are specifically designed for in-vitro studies, which are conducted outside of living organisms. In-vitro studies, derived from the Latin term "in glass," involve experiments performed in controlled laboratory settings using cells or tissues. It is important to note that these products are not categorized as medicines or drugs, and they have not received approval from the FDA for the prevention, treatment, or cure of any medical condition, ailment, or disease. We must emphasize that any form of bodily introduction of these products into humans or animals is strictly prohibited by law. It is essential to adhere to these guidelines to ensure compliance with legal and ethical standards in research and experimentation.