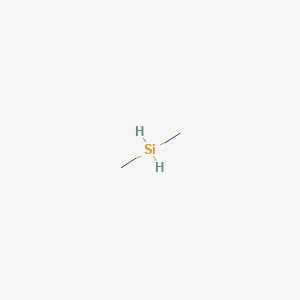
Dimethylsilane
Overview
Description
Dimethylsilane (C₂H₈Si; CAS RN: 1111-74-6) is a volatile organosilicon compound with a molecular mass of 60.17 g/mol . Key physical properties include a boiling point of -20°C and a melting point of -150°C, making it a low-viscosity liquid under standard conditions. Its simple structure—two methyl groups bonded to a silicon atom—renders it a versatile precursor in materials science, particularly in plasma-enhanced chemical vapor deposition (PECVD) for synthesizing silicon-based thin films .
Preparation Methods
Dimethylsilane can be synthesized through several methods. One common synthetic route involves the reduction of dimethyldichlorosilane with lithium aluminum hydride (LiAlH₄) or sodium borohydride (NaBH₄). Another method includes the reaction of dimethylchlorosilane with a Grignard reagent .
Industrial production of this compound often involves the hydrolysis of dimethyldichlorosilane in the presence of a base, followed by purification through distillation. This method is preferred due to its efficiency and scalability .
Chemical Reactions Analysis
Thermal Decomposition and Radical Pathways
DMS undergoes thermal decomposition in gas-phase reactions, producing intermediates like dimethylsilylene (Si(CH₃)₂) and methylsilene (CH₂=SiH(CH₃)) under high-temperature conditions (e.g., in hot-wire CVD processes). Key products include:
-
1,1,2,2-Tetramethyldisilane (TMDS) : Formed via silylene insertion into the Si–H bond of DMS .
-
1,3-Dimethyl-1,3-disilacyclobutane (DMDSCB) : Generated through self-dimerization of dimethylsilylene or methylsilene .
-
Trimethylsilane (TriMS) : Resulting from radical recombination .
Table 1: Thermal Decomposition Products of DMS
Product | Formation Mechanism | Dominant Conditions |
---|---|---|
TMDS | Silylene insertion | Low filament temperatures |
DMDSCB | Silylene/silene dimerization | Short reaction times |
TriMS | Radical recombination | High-temperature regimes |
H-Abstraction Reactions
DMS participates in H-abstraction reactions with radicals like H and CH₃, critical in combustion and oxidation processes. Computational studies reveal:
-
Lower Barrier Energies for Si–H vs. C–H Abstraction :
-
Abstraction from the secondary Si–H site in DMS has barriers of 4.3 kcal/mol (H attack) and 10.0 kcal/mol (CH₃ attack) , significantly lower than equivalent C–H sites in propane .
-
Primary C–H abstraction in DMS exhibits higher barriers (~10.8 kcal/mol for H attack) compared to propane (10.4 kcal/mol), attributed to electron-affinitive regions at carbon sites .
-
Table 2: Barrier Energies for H-Abstraction (kcal/mol)
Reaction Site | H Radical Attack | CH₃ Radical Attack |
---|---|---|
DMS (Secondary Si–H) | 4.3 | 10.0 |
Propane (Secondary C–H) | 8.2 | 12.8 |
DMS (Primary C–H) | 10.8 | 14.3 |
Hydrolysis and Condensation
While DMS is less reactive toward hydrolysis compared to chlorinated silanes, it can undergo controlled hydrolysis in the presence of catalysts:
-
Hydrogen Elimination : Liberates H₂ when exposed to Lewis acids (e.g., AlCl₃) or noble metals (Pt, Pd) .
-
Oligomerization : Forms polysilanes via sodium–potassium alloy-mediated polymerization .
Radical Chain Reactions in Combustion
DMS combustion involves radical propagation steps:
-
Chain Initiation : Si–H bond cleavage generates silyl radicals ((CH₃)₂SiH·) .
-
Chain Branching : Radicals react with O₂, forming siloxanes and SiO₂ particulates .
Table 3: Rate Constants for Key Combustion Reactions (cm³/mol·s)
Reaction | Temperature (K) | Rate Constant (k) |
---|---|---|
(CH₃)₂SiH₂ + H → (CH₃)₂SiH· + H₂ | 800–1200 | 2.1×10⁻¹⁰ – 5.6×10⁻⁹ |
(CH₃)₂SiH· + O₂ → SiO₂ + CH₃O· | 1000–1500 | 1.3×10⁻¹² – 3.8×10⁻¹¹ |
Catalytic Functionalization
DMS reacts with halides or alkoxides in the presence of Cu or Pt catalysts:
Scientific Research Applications
Chemical Synthesis
Dimethylsilane is extensively used as a reagent in organic synthesis. Its ability to participate in various chemical reactions makes it valuable for creating complex molecules in pharmaceuticals and agrochemicals.
Key Applications:
- Hydrosilylation Reactions: DMS can be used as a hydrosilane, facilitating the addition of silicon to unsaturated organic compounds, thus forming siloxanes.
- Reducing Agent: It acts as a mild reducing agent in organic transformations, particularly in the reduction of carbonyl compounds .
Silicone Production
DMS is crucial for producing silicone polymers, which are utilized in numerous applications due to their flexibility, thermal stability, and resistance to moisture.
Applications:
- Sealants and Adhesives: Silicones derived from DMS are employed in construction and automotive industries for sealing joints and bonding materials.
- Coatings: Silicone coatings provide protective barriers against environmental damage and enhance the durability of substrates .
Surface Modification
This compound is used to modify surfaces to improve properties such as adhesion and hydrophobicity. This is particularly relevant in the electronics industry.
Applications:
- Semiconductor Devices: DMS enhances the performance of semiconductor devices by modifying surface characteristics, which is vital for device efficiency .
- Nanotechnology: It aids in functionalizing nanoparticles, improving their stability and reactivity for applications in drug delivery systems .
Materials Science
In materials science, DMS contributes to the synthesis of novel materials with unique properties.
Applications:
- Polymer Synthesis: DMS is used to create high-performance polymers with tailored properties for specific applications .
- Nanocomposites: It plays a role in developing nanocomposites that exhibit improved mechanical properties and thermal stability .
Data Tables
Application Area | Specific Uses |
---|---|
Chemical Synthesis | Hydrosilylation, reduction reactions |
Silicone Production | Sealants, adhesives, coatings |
Surface Modification | Semiconductor devices, nanomaterials |
Materials Science | Polymer synthesis, nanocomposites |
Case Study 1: Use of this compound in Organic Synthesis
A study demonstrated that this compound effectively facilitated the hydrosilylation of alkenes to produce siloxanes with high yields. The reaction conditions were optimized for temperature and catalyst type, showcasing DMS's utility as a reagent in organic chemistry.
Case Study 2: this compound in Semiconductor Manufacturing
Research highlighted the role of this compound in enhancing the adhesion properties of thin films on semiconductor substrates. The modification improved device performance by reducing defects at interfaces.
Mechanism of Action
The mechanism of action of dimethylsilane involves its ability to undergo various chemical transformations. The compound can act as a reducing agent, donating electrons to other molecules. It can also participate in substitution reactions, where its silicon-hydrogen bonds are replaced by other functional groups. These reactions are facilitated by the presence of catalysts and specific reaction conditions .
Comparison with Similar Compounds
The reactivity, structural diversity, and applications of dimethylsilane are best contextualized by comparing it with analogous organosilicon compounds. Below is a detailed analysis:
Structural and Functional Group Comparisons
Di(phenylethynyl)this compound
- Molecular Formula : C₁₈H₁₆Si
- Key Features : Incorporates phenylethynyl substituents, which enhance π-conjugation and rigidity.
- Applications : Serves as a model compound for studying substituent effects on silicon-centered bonding via X-ray crystallography and multinuclear NMR (¹³C, ²⁹Si) .
- Contrast with this compound : The bulky phenylethynyl groups increase steric hindrance and thermal stability compared to this compound’s compact methyl groups.
Bis(dimethylamino)this compound (BDMADMS)
- Molecular Formula : C₆H₁₈N₂Si₂
- Key Features: Contains dimethylamino (-N(CH₃)₂) groups, which introduce nitrogen into deposited films.
- Applications : Used in PECVD to synthesize silicon carbonitride (SiCN) films with tailored hardness (2.5–16 GPa) and Young’s modulus (43–187 GPa) .
- Contrast with this compound : Nitrogen incorporation in BDMADMS-derived films improves mechanical and gas-separation properties, unlike pure silicon-carbon films from this compound .
Physicochemical and Application-Based Comparisons
Thermal Stability and Reactivity
- This compound : Highly reactive due to Si–H bonds, enabling rapid decomposition in PECVD to form SiC or SiCH networks .
- Diisobutyl this compound : Features branched isobutyl groups, reducing reactivity but enhancing thermal stability for low-porosity SiCH films with low dielectric constants (k ≈ 2.5) .
Data Table: Comparative Properties of Selected Organosilicon Compounds
Substituent Effects on Material Properties
- Methyl vs. Amino Groups: this compound’s methyl groups yield hydrophobic SiCH films, while BDMADMS’s amino groups facilitate nitrogen-rich SiCN films with enhanced mechanical strength .
- Vinyl vs. Ethynyl Groups : Vinyl-substituted silanes (e.g., dimethyldivinylsilane) enable crosslinking in polymers, whereas ethynyl groups in di(phenylethynyl)this compound promote rigidity and conjugation .
Research Findings and Trends
PECVD Applications : this compound and its derivatives are critical for low-k dielectric films in microelectronics. For example, diisobutyl this compound enables SiCH films with Si–C₂H₄–Si networks, achieving k-values < 2.5 .
Biological Relevance : AzhepSi demonstrates that this compound derivatives can be engineered for targeted biological interactions, a niche unexplored for pure this compound .
Structural Insights: NMR studies reveal that this compound’s δ(¹³C) shifts differ markedly from strained metallocenophanes, highlighting electronic environment variations .
Q & A
Basic Research Questions
Q. What are the optimal synthesis conditions for dimethylsilane in laboratory settings, and how can purity be validated?
- Methodological Guidance: this compound synthesis typically involves Grignard reactions or catalytic reduction of chlorosilanes. To ensure high purity, employ distillation under inert atmospheres and monitor reaction parameters (temperature, pressure, and catalyst loading) rigorously. Validate purity using gas chromatography-mass spectrometry (GC-MS) and nuclear magnetic resonance (NMR) spectroscopy, ensuring alignment with known spectral databases. Report detailed protocols, including inert gas purging and quenching steps, to enable reproducibility .
Q. How should researchers document this compound experiments to ensure reproducibility and compliance with safety standards?
- Methodological Guidance: Adhere to FAIR data principles (Findable, Accessible, Interoperable, Reusable) by depositing raw datasets in repositories like Zenodo or ICPSR. Include metadata on equipment calibration, batch numbers of chemicals, and environmental conditions. Publish detailed supplemental materials with step-by-step protocols, referencing institutional safety committee approvals .
Properties
IUPAC Name |
dimethylsilane | |
---|---|---|
Details | Computed by LexiChem 2.6.6 (PubChem release 2019.06.18) | |
Source | PubChem | |
URL | https://pubchem.ncbi.nlm.nih.gov | |
Description | Data deposited in or computed by PubChem | |
InChI |
InChI=1S/C2H8Si/c1-3-2/h3H2,1-2H3 | |
Details | Computed by InChI 1.0.5 (PubChem release 2019.06.18) | |
Source | PubChem | |
URL | https://pubchem.ncbi.nlm.nih.gov | |
Description | Data deposited in or computed by PubChem | |
InChI Key |
UBHZUDXTHNMNLD-UHFFFAOYSA-N | |
Details | Computed by InChI 1.0.5 (PubChem release 2019.06.18) | |
Source | PubChem | |
URL | https://pubchem.ncbi.nlm.nih.gov | |
Description | Data deposited in or computed by PubChem | |
Canonical SMILES |
C[SiH2]C | |
Details | Computed by OEChem 2.1.5 (PubChem release 2019.06.18) | |
Source | PubChem | |
URL | https://pubchem.ncbi.nlm.nih.gov | |
Description | Data deposited in or computed by PubChem | |
Molecular Formula |
C2H8Si | |
Details | Computed by PubChem 2.1 (PubChem release 2019.06.18) | |
Source | PubChem | |
URL | https://pubchem.ncbi.nlm.nih.gov | |
Description | Data deposited in or computed by PubChem | |
Related CAS |
110477-50-4 | |
Details | Compound: Silane, dimethyl-, homopolymer | |
Record name | Silane, dimethyl-, homopolymer | |
Source | CAS Common Chemistry | |
URL | https://commonchemistry.cas.org/detail?cas_rn=110477-50-4 | |
Description | CAS Common Chemistry is an open community resource for accessing chemical information. Nearly 500,000 chemical substances from CAS REGISTRY cover areas of community interest, including common and frequently regulated chemicals, and those relevant to high school and undergraduate chemistry classes. This chemical information, curated by our expert scientists, is provided in alignment with our mission as a division of the American Chemical Society. | |
Explanation | The data from CAS Common Chemistry is provided under a CC-BY-NC 4.0 license, unless otherwise stated. | |
Molecular Weight |
60.17 g/mol | |
Details | Computed by PubChem 2.1 (PubChem release 2021.05.07) | |
Source | PubChem | |
URL | https://pubchem.ncbi.nlm.nih.gov | |
Description | Data deposited in or computed by PubChem | |
CAS No. |
1111-74-6 | |
Record name | Dimethylsilane | |
Source | CAS Common Chemistry | |
URL | https://commonchemistry.cas.org/detail?cas_rn=1111-74-6 | |
Description | CAS Common Chemistry is an open community resource for accessing chemical information. Nearly 500,000 chemical substances from CAS REGISTRY cover areas of community interest, including common and frequently regulated chemicals, and those relevant to high school and undergraduate chemistry classes. This chemical information, curated by our expert scientists, is provided in alignment with our mission as a division of the American Chemical Society. | |
Explanation | The data from CAS Common Chemistry is provided under a CC-BY-NC 4.0 license, unless otherwise stated. | |
Retrosynthesis Analysis
AI-Powered Synthesis Planning: Our tool employs the Template_relevance Pistachio, Template_relevance Bkms_metabolic, Template_relevance Pistachio_ringbreaker, Template_relevance Reaxys, Template_relevance Reaxys_biocatalysis model, leveraging a vast database of chemical reactions to predict feasible synthetic routes.
One-Step Synthesis Focus: Specifically designed for one-step synthesis, it provides concise and direct routes for your target compounds, streamlining the synthesis process.
Accurate Predictions: Utilizing the extensive PISTACHIO, BKMS_METABOLIC, PISTACHIO_RINGBREAKER, REAXYS, REAXYS_BIOCATALYSIS database, our tool offers high-accuracy predictions, reflecting the latest in chemical research and data.
Strategy Settings
Precursor scoring | Relevance Heuristic |
---|---|
Min. plausibility | 0.01 |
Model | Template_relevance |
Template Set | Pistachio/Bkms_metabolic/Pistachio_ringbreaker/Reaxys/Reaxys_biocatalysis |
Top-N result to add to graph | 6 |
Feasible Synthetic Routes
Disclaimer and Information on In-Vitro Research Products
Please be aware that all articles and product information presented on BenchChem are intended solely for informational purposes. The products available for purchase on BenchChem are specifically designed for in-vitro studies, which are conducted outside of living organisms. In-vitro studies, derived from the Latin term "in glass," involve experiments performed in controlled laboratory settings using cells or tissues. It is important to note that these products are not categorized as medicines or drugs, and they have not received approval from the FDA for the prevention, treatment, or cure of any medical condition, ailment, or disease. We must emphasize that any form of bodily introduction of these products into humans or animals is strictly prohibited by law. It is essential to adhere to these guidelines to ensure compliance with legal and ethical standards in research and experimentation.