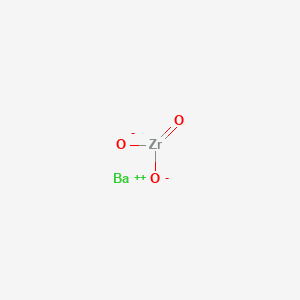
Barium zirconate
Overview
Description
Barium zirconate (BaZrO₃) is a perovskite oxide with a cubic crystal structure (lattice parameter a = 4.192 Å in bulk) at room temperature . Unlike ferroelectric BaTiO₃, BaZrO₃ is paraelectric in its free state, lacking spontaneous polarization . However, its properties are highly tunable in engineered structures. For example, in BaZrO₃/BaTiO₃ superlattices, mechanical stresses from adjacent layers induce polarization in BaZrO₃, enabling ferroelectric behavior with a Curie temperature (TC) of ~393°C—significantly higher than pure BaTiO₃ films or bulk (~120°C) . These superlattices exhibit exceptional dielectric constants (ε > 10,000) and low dielectric loss (tan δ ≈ 5 × 10⁻³), making them promising for integrated electronics .
BaZrO₃ is also notable for proton conductivity when doped (e.g., with yttrium), though its dense ceramic fabrication historically required advanced sintering techniques like solid-state reactive sintering with NiO or ZnO additives . Its stability under harsh environments (e.g., CO₂, H₂O) contrasts with chemically unstable analogs like BaCeO₃ .
Preparation Methods
Synthetic Routes and Reaction Conditions
Barium zirconate can be synthesized using several methods, including solid-state reactions, sol-gel processes, and hydrothermal synthesis.
Solid-State Reactions: This method involves mixing barium carbonate and zirconium dioxide powders, followed by calcination at high temperatures (around 1400°C) to form this compound.
Sol-Gel Process: In this method, barium acetate and zirconium oxychloride are dissolved in a solvent to form a gel. The gel is then dried and calcined to obtain this compound.
Hydrothermal Synthesis: This involves reacting barium hydroxide and zirconium nitrate in a high-pressure, high-temperature aqueous solution to produce this compound nanoparticles.
Industrial Production Methods
Industrial production of this compound typically employs the solid-state reaction method due to its simplicity and scalability. The process involves precise control of temperature and reaction time to ensure high purity and crystallinity of the final product.
Chemical Reactions Analysis
Barium zirconate undergoes various chemical reactions, including:
Oxidation: this compound can react with oxygen at high temperatures to form this compound oxide.
Reduction: Under reducing conditions, this compound can be reduced to barium oxide and zirconium dioxide.
Common reagents used in these reactions include oxygen, hydrogen, and various metal salts. The major products formed depend on the specific reaction conditions and reagents used.
Scientific Research Applications
Electrochemical Applications
1.1 Proton Conductors
Barium zirconate is extensively studied as a proton-conducting electrolyte in fuel cells and electrochemical devices. Its high ionic conductivity at elevated temperatures makes it suitable for solid oxide fuel cells (SOFCs) and hydrogen fuel cells. Recent reviews highlight the advancements in this compound proton conductors, focusing on their synthesis methods, structural properties, and performance metrics in electrochemical applications .
Parameter | Value |
---|---|
Ionic Conductivity | Up to at 600°C |
Operating Temperature | 600°C - 800°C |
Stability | High under reducing atmospheres |
1.2 Hydrogen Isotope Separation
This compound also shows promise in hydrogen isotope separation technologies due to its selective proton conduction properties. This application is particularly relevant for nuclear fusion research and hydrogen production processes .
Dental Materials
This compound is utilized as a radiopacifier in dental materials, specifically in mineral trioxide aggregates (MTAs) used for endodontic treatments. Studies have demonstrated that BaZrO₃ enhances the radiopacity of MTAs, making them more visible during X-ray examinations. It has been shown that this compound can improve the mechanical properties and setting times of these materials .
Property | MTA with BaZrO₃ | Commercial MTA |
---|---|---|
Radiopacity (mmAl) | 3.60 ± 0.22 | Varies |
Diametral Tensile Strength (MPa) | 3.02 ± 0.18 | Varies |
Setting Time (minutes) | Significantly shorter | Varies |
Electronic Components
3.1 Dielectric Materials
This compound is widely used in dielectric applications due to its high dielectric constant and low loss tangent. It is often incorporated into composite materials with polymers like poly(vinylidene fluoride) (PVDF) to enhance their electrical properties, making them suitable for sensors, actuators, and transducers .
3.2 Thin Films for Photonic Devices
Research has shown that BaZrO₃ thin films exhibit excellent electro-optic properties, making them suitable for photonic devices and modulators used in telecommunications . The integration of this compound into photonic crystal structures has been explored to improve optical performance across various wavelengths.
Summary of Key Findings
This compound's multifaceted applications stem from its unique physical and chemical properties. Its role as a proton conductor in electrochemical devices highlights its potential in energy technologies, while its use in dental materials emphasizes its importance in healthcare applications. Additionally, its incorporation into electronic components showcases its versatility across different industries.
Case Studies
- Electrochemical Devices: A study on yttrium-doped this compound revealed enhanced conductivity and stability under operational conditions typical for SOFCs .
- Dental Applications: Research demonstrated that incorporating this compound into MTAs significantly improved their mechanical strength and radiopacity compared to traditional materials .
Mechanism of Action
The mechanism by which barium zirconate exerts its effects is primarily through its ionic conductivity and stability. In fuel cells, for example, this compound facilitates the transport of oxygen ions, enhancing the efficiency of the cell. The molecular targets and pathways involved include the lattice structure of this compound, which allows for the movement of ions through its crystal lattice .
Comparison with Similar Compounds
Structural Analogs: Perovskite Oxides
Barium Titanate (BaTiO₃)
Key Differences :
- BaTiO₃ exhibits intrinsic ferroelectricity, while BaZrO₃ requires structural engineering (e.g., superlattices) to induce polarization.
- Superlattices combine high TC and ε, outperforming individual components .
Strontium Titanate (SrTiO₃)
- Cubic perovskite with higher dielectric tunability but lower ε (~300 at room temp) compared to BaZrO₃-based superlattices .
- Used in tunable microwave devices, whereas BaZrO₃ excels in high-temperature stability .
Functional Analogs: Proton Conductors and Ferroelectrics
Barium Cerate (BaCeO₃)
Property | BaCeO₃ | BaZrO₃ |
---|---|---|
Proton Conductivity (S/cm) | ~10⁻² (600°C) | ~10⁻³ (600°C) |
Chemical Stability | Poor (degraded by CO₂/H₂O) | Excellent |
Sintering | Easier | Requires additives (NiO/ZnO) |
Trade-offs : BaCeO₃ offers higher conductivity but poor durability, limiting its use in fuel cells. Doped BaZrO₃ (e.g., Y-BaZrO₃) balances stability and moderate conductivity .
Lead Zirconate Titanate (PZT)
- Ferroelectric workhorse with high ε (~2,000) but contains toxic lead .
- BaZrO₃-based superlattices provide a lead-free alternative with comparable ε and higher TC .
Composite and Engineered Systems
BaZrO₃/BaTiO₃ Superlattices
- Structure : 32 alternating layers (6.65 nm BaZrO₃ / 6.67 nm BaTiO₃) on MgO substrates .
- Performance :
- Mechanism : Strain-induced polarization in BaZrO₃ and elastic coupling between layers enhance TC and ε .
Doped BaZrO₃ Composites
- Yttrium-Doped BaZrO₃ (BZY) : Ionic transport number ~0.9 at 600°C, suitable for fuel cells .
- ZnO-Modified BaZrO₃: 93% densification at 1,300°C, though conductivity slightly reduced .
Data Tables
Table 1: Dielectric and Structural Properties
Table 2: Proton Conductors
Compound | Conductivity (S/cm, 600°C) | Stability | Sintering Aid |
---|---|---|---|
Y-BaZrO₃ | ~10⁻³ | Excellent | NiO, ZnO |
BaCeO₃ | ~10⁻² | Poor | None |
Key Research Findings
- Superlattice Engineering : BaZrO₃/BaTiO₃ superlattices achieve ε >10,000 and TC ~393°C, enabling integration into low-voltage silicon devices .
- Sintering Advances: Solid-state reactive sintering with ZnO/NiO allows dense BaZrO₃ ceramics (>93% density) for fuel cells .
- Stability vs. Conductivity : BaZrO₃ sacrifices some proton conductivity for unmatched chemical robustness compared to BaCeO₃ .
Biological Activity
Barium zirconate (BaZrO₃) is a perovskite-type oxide that has garnered attention for its potential biological applications, particularly in antimicrobial activity and biocompatibility. This article synthesizes current research findings on the biological activity of this compound, focusing on its antibacterial properties, potential applications in biomedical fields, and relevant case studies.
Antibacterial Properties
Recent studies have demonstrated that this compound exhibits significant antibacterial activity against both gram-positive and gram-negative bacteria. The antibacterial properties are particularly notable in its nanoparticle form, specifically this compound titanate (BZT), which combines this compound with titanium dioxide.
Key Findings
-
Antibacterial Efficacy :
- BZT nanoparticles were synthesized using the sol-gel method and characterized to confirm their perovskite structure. These nanoparticles demonstrated effective antibacterial activity against Staphylococcus aureus, Micrococcus luteus, Escherichia coli, and Klebsiella pneumoniae.
- Minimum inhibitory concentration (MIC) values were determined as follows:
- E. coli: 2.3 μg/mL
- K. pneumoniae: 7.3 μg/mL
- M. luteus: 3 μg/mL
- S. aureus: 12 μg/mL
- Minimum bactericidal concentration (MBC) values were also established, indicating the concentrations required to kill the bacteria after exposure to BZT nanoparticles .
-
Mechanism of Action :
- The exact mechanism by which this compound exerts its antibacterial effects is still under investigation. However, it is believed that the nanoparticles disrupt bacterial cell membranes and interfere with cellular processes, leading to cell death.
Biocompatibility Studies
Biocompatibility is crucial for materials intended for medical applications. Studies have evaluated the hemolytic activity of BZT nanoparticles, indicating that while they possess antibacterial properties, their hemolytic activity remains relatively low, suggesting potential safety for use in biological systems .
Case Study 1: Synthesis and Characterization of BZT Nanoparticles
A study focused on the synthesis of BZT nanoparticles through a sol-gel method at a calcination temperature of 1000 °C. The resulting nanoparticles exhibited a single-phase perovskite structure confirmed by X-ray diffraction analysis. The study highlighted their effective antibacterial properties against selected bacterial strains through radial diffusion assays .
Case Study 2: Application in Biomedical Fields
Another investigation explored the application of this compound in drug delivery systems due to its biocompatibility and antimicrobial properties. The study suggested that BZT could serve as a carrier for antibiotic drugs, enhancing their efficacy while reducing dosages required for treatment .
Comparative Table of Antibacterial Activity
Bacterial Strain | MIC (μg/mL) | MBC (μg/mL) |
---|---|---|
Escherichia coli | 2.3 | 2.3 |
Klebsiella pneumoniae | 7.3 | 14 |
Micrococcus luteus | 3 | 3 |
Staphylococcus aureus | 12 | 18 |
Q & A
Basic Research Questions
Q. What are the standard synthesis protocols for barium zirconate, and how do they influence material purity and crystallinity?
this compound is typically synthesized via solid-state reactions, sol-gel methods, or co-precipitation. Solid-state reactions involve high-temperature calcination (≥1300°C) of BaCO₃ and ZrO₂ precursors, but may introduce impurities due to incomplete mixing. Sol-gel methods, using alkoxide precursors (e.g., zirconium isopropoxide), enable better stoichiometric control and lower sintering temperatures (~1000°C), improving phase purity. Post-synthesis characterization via X-ray diffraction (XRD) and scanning electron microscopy (SEM) is critical to verify cubic perovskite structure and grain homogeneity .
Q. How do researchers characterize the local and long-range structural distortions in doped this compound?
High-resolution XRD resolves long-range tetragonal distortions in yttrium-doped BaZrO₃ (BZY), while extended X-ray absorption fine structure (EXAFS) probes short-range coordination environments around Zr⁴⁺ and Y³⁺. Raman spectroscopy complements these by identifying symmetry-breaking vibrational modes caused by dopant-induced lattice strain. For example, Y³⁅ doping increases local distortions due to its larger ionic radius compared to Zr⁴⁺, which correlates with reduced proton mobility .
Q. What analytical methods are used to assess proton conductivity in this compound-based electrolytes?
Electrochemical impedance spectroscopy (EIS) is the primary method, measuring conductivity under controlled humidity and temperature (e.g., 400–700°C). Hydration conditions must be standardized, as proton conduction relies on hydroxyl ion mobility. Data analysis typically involves fitting impedance arcs to equivalent circuits to separate bulk, grain boundary, and electrode contributions .
Advanced Research Questions
Q. How do oxygen vacancies and dopant-proton interactions influence proton conduction pathways in yttrium-doped this compound?
Oxygen vacancies (VO••) in BZY act as proton traps, localizing near Y³⁺ dopants due to electrostatic attraction. Kinetic Monte Carlo (KMC) simulations reveal that proton migration barriers increase from 0.39 eV to 0.45 eV as proton concentration rises, suggesting vacancy-proton repulsion limits conductivity. Machine learning-trained potential energy surfaces (PES) derived from ab initio data can model low-energy proton paths in Y:BaZrO₃ systems with vacancies, aiding in optimizing dopant-vacancy ratios .
Q. What computational strategies address discrepancies between experimental and theoretical proton conduction barriers in scandium-doped this compound?
Density functional theory (DFT) often underestimates proton migration barriers due to approximations in exchange-correlation functionals. Hybrid functionals (e.g., HSE06) improve accuracy but are computationally costly. Machine learning force fields trained on DFT datasets enable larger-scale molecular dynamics simulations, capturing dynamic lattice distortions and validating against experimental barriers (e.g., 0.50 eV for Sc-doped BaZrO₃ vs. 0.52 eV in simulations) .
Q. How can researchers reconcile the trade-off between proton conductivity and chemical stability in this compound vs. cerate systems?
this compound (BaZrO₃) exhibits superior stability under CO₂/H₂O environments but lower conductivity (~10⁻³ S/cm at 600°C) compared to barium cerate (BaCeO₃, ~10⁻² S/cm). Composite electrolytes (e.g., BaZrO₃–carbonate mixtures) leverage interfacial proton transport mechanisms to enhance conductivity while retaining stability. Systematic doping (e.g., Y/Sc co-doping) or grain boundary engineering (e.g., ZnO sintering aids) further mitigates this trade-off .
Q. What experimental designs resolve contradictions in dopant solubility limits and lattice strain reports for this compound?
Contradictions arise from variations in synthesis conditions (e.g., cooling rates, precursor homogeneity) and characterization techniques. High-throughput combinatorial synthesis coupled with spatially resolved XRD/EXAFS can map dopant solubility thresholds. For instance, Y³⁺ solubility in BaZrO₃ is limited to ~20% due to strain from ionic radius mismatch, validated by Raman peak broadening and HR-XRD lattice parameter deviations .
Q. Methodological Guidance
-
Addressing Data Variability in Conductivity Measurements:
Normalize EIS data to sample geometry (e.g., thickness/area) and account for interfacial polarization effects using guard-ring electrodes. Replicate measurements under identical hydration conditions to isolate material-specific trends from experimental artifacts . -
Optimizing Doping Strategies:
Use defect chemistry models to predict dopant-vacancy equilibria. For example, Kröger-Vink notation can describe charge compensation mechanisms (e.g., ) and guide co-doping approaches to minimize trapping . -
Validating Computational Models:
Cross-check machine learning-derived PES with nudged elastic band (NEB) calculations for key proton migration paths. Benchmark against experimental activation energies and isotopic tracer diffusion data .
Properties
IUPAC Name |
barium(2+);dioxido(oxo)zirconium | |
---|---|---|
Details | Computed by Lexichem TK 2.7.0 (PubChem release 2021.05.07) | |
Source | PubChem | |
URL | https://pubchem.ncbi.nlm.nih.gov | |
Description | Data deposited in or computed by PubChem | |
InChI |
InChI=1S/Ba.3O.Zr/q+2;;2*-1; | |
Details | Computed by InChI 1.0.6 (PubChem release 2021.05.07) | |
Source | PubChem | |
URL | https://pubchem.ncbi.nlm.nih.gov | |
Description | Data deposited in or computed by PubChem | |
InChI Key |
DQBAOWPVHRWLJC-UHFFFAOYSA-N | |
Details | Computed by InChI 1.0.6 (PubChem release 2021.05.07) | |
Source | PubChem | |
URL | https://pubchem.ncbi.nlm.nih.gov | |
Description | Data deposited in or computed by PubChem | |
Canonical SMILES |
[O-][Zr](=O)[O-].[Ba+2] | |
Details | Computed by OEChem 2.3.0 (PubChem release 2021.05.07) | |
Source | PubChem | |
URL | https://pubchem.ncbi.nlm.nih.gov | |
Description | Data deposited in or computed by PubChem | |
Molecular Formula |
BaZrO3, BaO3Zr | |
Details | Computed by PubChem 2.1 (PubChem release 2021.05.07) | |
Record name | barium zirconate | |
Source | Wikipedia | |
URL | https://en.wikipedia.org/wiki/Dictionary_of_chemical_formulas | |
Description | Chemical information link to Wikipedia. | |
Details | Computed by PubChem 2.1 (PubChem release 2021.05.07) | |
Source | PubChem | |
URL | https://pubchem.ncbi.nlm.nih.gov | |
Description | Data deposited in or computed by PubChem | |
DSSTOX Substance ID |
DTXSID90893160 | |
Record name | Barium zirconate (BaZrO3) | |
Source | EPA DSSTox | |
URL | https://comptox.epa.gov/dashboard/DTXSID90893160 | |
Description | DSSTox provides a high quality public chemistry resource for supporting improved predictive toxicology. | |
Molecular Weight |
276.55 g/mol | |
Details | Computed by PubChem 2.1 (PubChem release 2021.05.07) | |
Source | PubChem | |
URL | https://pubchem.ncbi.nlm.nih.gov | |
Description | Data deposited in or computed by PubChem | |
Physical Description |
Off-white to gray powder; [MSDSonline] Solubility--no data found; | |
Record name | Barium zirconate | |
Source | Haz-Map, Information on Hazardous Chemicals and Occupational Diseases | |
URL | https://haz-map.com/Agents/7989 | |
Description | Haz-Map® is an occupational health database designed for health and safety professionals and for consumers seeking information about the adverse effects of workplace exposures to chemical and biological agents. | |
Explanation | Copyright (c) 2022 Haz-Map(R). All rights reserved. Unless otherwise indicated, all materials from Haz-Map are copyrighted by Haz-Map(R). No part of these materials, either text or image may be used for any purpose other than for personal use. Therefore, reproduction, modification, storage in a retrieval system or retransmission, in any form or by any means, electronic, mechanical or otherwise, for reasons other than personal use, is strictly prohibited without prior written permission. | |
CAS No. |
12009-21-1 | |
Record name | Barium zirconate (BaZrO3) | |
Source | EPA DSSTox | |
URL | https://comptox.epa.gov/dashboard/DTXSID90893160 | |
Description | DSSTox provides a high quality public chemistry resource for supporting improved predictive toxicology. | |
Disclaimer and Information on In-Vitro Research Products
Please be aware that all articles and product information presented on BenchChem are intended solely for informational purposes. The products available for purchase on BenchChem are specifically designed for in-vitro studies, which are conducted outside of living organisms. In-vitro studies, derived from the Latin term "in glass," involve experiments performed in controlled laboratory settings using cells or tissues. It is important to note that these products are not categorized as medicines or drugs, and they have not received approval from the FDA for the prevention, treatment, or cure of any medical condition, ailment, or disease. We must emphasize that any form of bodily introduction of these products into humans or animals is strictly prohibited by law. It is essential to adhere to these guidelines to ensure compliance with legal and ethical standards in research and experimentation.