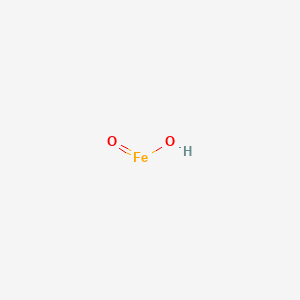
hydroxy(oxo)iron
Overview
Description
Goethite, with the chemical formula Fe(OH)O, is a widespread iron oxide mineral and a common ingredient of iron rust. It was named in 1806 after Johann Wolfgang von Goethe, a German poet and philosopher with a keen interest in minerals . Goethite is typically formed under oxidizing conditions as a weathering product of iron minerals such as pyrite and magnetite . It is also a primary precipitate in marine and meteoric waters and accumulates in springs and marshes .
Mechanism of Action
Target of Action
Hydroxy(oxo)iron, also known as Goethite or Lepidocrocite, primarily targets erythroid precursor cells . These cells are crucial in the production of red blood cells, which are responsible for transporting oxygen throughout the body .
Mode of Action
The mode of action of this compound involves an initial hydrogen atom abstraction from the substrate by high-valent iron-oxo species . This generates a substrate radical and a reduced iron hydroxide . This type of reactivity is characteristic of radicals, species that have unpaired electrons .
Biochemical Pathways
The biochemical pathways affected by this compound are diverse. The Fe(II)/2-oxoglutarate-dependent oxygenases catalyze a wide array of biochemical reactions . These enzymes function in biosynthesis and catabolism of cellular metabolites, including antibiotics and signaling molecules .
Pharmacokinetics
The pharmacokinetics of this compound involve its administration either intravenously in the form of polynuclear iron(III)-hydroxide complexes with carbohydrate ligands or orally as iron(II) (ferrous) salts or iron(III) (ferric) complexes . High oral iron doses or rapid release of iron from intravenous iron preparations can saturate the iron transport system, resulting in oxidative stress with adverse clinical and subclinical consequences .
Result of Action
The result of this compound’s action is the formation of new erythrocytes, or red blood cells . The iron is incorporated into hemoglobin as the cells mature into red blood cells . This process is crucial for the body’s ability to transport oxygen.
Action Environment
The action of this compound can be influenced by various environmental factors. For instance, the efficiency of absorption depends on the salt form, the amount administered, the dosing regimen, and the size of iron stores . Furthermore, the stability of the iron complex is directly related to the molecular weight and the stability of the complex .
Biochemical Analysis
Biochemical Properties
Hydroxy(oxo)iron interacts with various enzymes, proteins, and other biomolecules. The reactivity of the metal oxo and hydroxo moieties in different oxidation processes, including hydrogen atom transfer, oxygen atom transfer, and electron transfer, has been discussed . Iron and manganese ions with terminal oxo and hydroxo ligands are key intermediates in several synthetic and biochemical catalytic cycles .
Cellular Effects
The effects of this compound on various types of cells and cellular processes are complex and multifaceted. It influences cell function, including any impact on cell signaling pathways, gene expression, and cellular metabolism
Molecular Mechanism
This compound exerts its effects at the molecular level through various mechanisms. It involves binding interactions with biomolecules, enzyme inhibition or activation, and changes in gene expression
Temporal Effects in Laboratory Settings
The effects of this compound over time in laboratory settings are complex. It involves the product’s stability, degradation, and any long-term effects on cellular function observed in in vitro or in vivo studies
Metabolic Pathways
This compound is involved in various metabolic pathways. It interacts with enzymes or cofactors and can affect metabolic flux or metabolite levels
Transport and Distribution
This compound is transported and distributed within cells and tissues. It can interact with transporters or binding proteins, and it can affect its localization or accumulation
Preparation Methods
Synthetic Routes and Reaction Conditions
Goethite can be synthesized through various methods. One common method involves the aging of iron(III) perchlorate solutions at room temperature, which precipitates goethite initially and produces hematite later with prolonged aging . Another method involves the simultaneous addition of iron(III) nitrate and a precipitating agent like ammonium hydroxide to ultrapure water, resulting in a pH of 10 . The system is continuously agitated to form goethite .
Industrial Production Methods
In industrial settings, goethite is often produced as a byproduct of the hydrometallurgical processing of iron ores. The process involves the oxidation of ferrous iron in acidic solutions, leading to the precipitation of goethite . This method is commonly used in the production of pigments and as a precursor for other iron oxides .
Chemical Reactions Analysis
Types of Reactions
Goethite undergoes various chemical reactions, including oxidation, reduction, and substitution. It is commonly involved in redox reactions due to its iron content .
Common Reagents and Conditions
Oxidation: Goethite can be oxidized to form hematite (Fe2O3) under high-temperature conditions.
Reduction: Under reducing conditions, goethite can be reduced to form magnetite (Fe3O4) or even metallic iron.
Substitution: Goethite can undergo substitution reactions where iron is replaced by other cations such as aluminum or nickel.
Major Products
Oxidation: Hematite (Fe2O3)
Reduction: Magnetite (Fe3O4), Metallic Iron
Substitution: Various substituted goethite forms with different cations.
Scientific Research Applications
Goethite has a wide range of scientific research applications:
Chemistry: Used as a catalyst in various chemical reactions, including the oxidation of organic compounds.
Biology: Studied for its role in iron cycling and its interactions with biological systems.
Medicine: Investigated for its potential use in drug delivery systems due to its biocompatibility.
Industry: Used as a pigment in paints and coatings, and as a precursor for other iron oxides.
Comparison with Similar Compounds
Similar Compounds
Lepidocrocite (γ-FeO(OH)): Similar chemical composition but different crystal structure.
Hematite (Fe2O3): Formed from the dehydration of goethite.
Magnetite (Fe3O4): Formed from the reduction of goethite.
Uniqueness
Goethite is unique due to its widespread occurrence and its role in various geological and environmental processes. Its ability to undergo various chemical reactions and its applications in different fields make it a versatile and valuable compound .
Properties
IUPAC Name |
hydroxy(oxo)iron | |
---|---|---|
Details | Computed by Lexichem TK 2.7.0 (PubChem release 2021.05.07) | |
Source | PubChem | |
URL | https://pubchem.ncbi.nlm.nih.gov | |
Description | Data deposited in or computed by PubChem | |
InChI |
InChI=1S/Fe.H2O.O/h;1H2;/q+1;;/p-1 | |
Details | Computed by InChI 1.0.6 (PubChem release 2021.05.07) | |
Source | PubChem | |
URL | https://pubchem.ncbi.nlm.nih.gov | |
Description | Data deposited in or computed by PubChem | |
InChI Key |
AEIXRCIKZIZYPM-UHFFFAOYSA-M | |
Details | Computed by InChI 1.0.6 (PubChem release 2021.05.07) | |
Source | PubChem | |
URL | https://pubchem.ncbi.nlm.nih.gov | |
Description | Data deposited in or computed by PubChem | |
Canonical SMILES |
O[Fe]=O | |
Details | Computed by OEChem 2.3.0 (PubChem release 2021.05.07) | |
Source | PubChem | |
URL | https://pubchem.ncbi.nlm.nih.gov | |
Description | Data deposited in or computed by PubChem | |
Molecular Formula |
FeO2H, FeHO2 | |
Details | Computed by PubChem 2.1 (PubChem release 2021.05.07) | |
Record name | goethite | |
Source | Wikipedia | |
URL | https://en.wikipedia.org/wiki/Goethite | |
Description | Chemical information link to Wikipedia. | |
Details | Computed by PubChem 2.1 (PubChem release 2021.05.07) | |
Source | PubChem | |
URL | https://pubchem.ncbi.nlm.nih.gov | |
Description | Data deposited in or computed by PubChem | |
DSSTOX Substance ID |
DTXSID801318339 | |
Record name | Akaganeite | |
Source | EPA DSSTox | |
URL | https://comptox.epa.gov/dashboard/DTXSID801318339 | |
Description | DSSTox provides a high quality public chemistry resource for supporting improved predictive toxicology. | |
Molecular Weight |
88.85 g/mol | |
Details | Computed by PubChem 2.1 (PubChem release 2021.05.07) | |
Source | PubChem | |
URL | https://pubchem.ncbi.nlm.nih.gov | |
Description | Data deposited in or computed by PubChem | |
Physical Description |
Yellow-brown to dark brown mineral; [Merck Index] Yellow-brown odorless powder; Insoluble in water (< 0.5%); [Prince Agri MSDS] | |
Record name | Goethite | |
Source | Haz-Map, Information on Hazardous Chemicals and Occupational Diseases | |
URL | https://haz-map.com/Agents/20975 | |
Description | Haz-Map® is an occupational health database designed for health and safety professionals and for consumers seeking information about the adverse effects of workplace exposures to chemical and biological agents. | |
Explanation | Copyright (c) 2022 Haz-Map(R). All rights reserved. Unless otherwise indicated, all materials from Haz-Map are copyrighted by Haz-Map(R). No part of these materials, either text or image may be used for any purpose other than for personal use. Therefore, reproduction, modification, storage in a retrieval system or retransmission, in any form or by any means, electronic, mechanical or otherwise, for reasons other than personal use, is strictly prohibited without prior written permission. | |
CAS No. |
12134-57-5, 1310-14-1, 20344-49-4, 12022-37-6 | |
Record name | Akaganeite | |
Source | CAS Common Chemistry | |
URL | https://commonchemistry.cas.org/detail?cas_rn=12134-57-5 | |
Description | CAS Common Chemistry is an open community resource for accessing chemical information. Nearly 500,000 chemical substances from CAS REGISTRY cover areas of community interest, including common and frequently regulated chemicals, and those relevant to high school and undergraduate chemistry classes. This chemical information, curated by our expert scientists, is provided in alignment with our mission as a division of the American Chemical Society. | |
Explanation | The data from CAS Common Chemistry is provided under a CC-BY-NC 4.0 license, unless otherwise stated. | |
Record name | Goethite | |
Source | CAS Common Chemistry | |
URL | https://commonchemistry.cas.org/detail?cas_rn=1310-14-1 | |
Description | CAS Common Chemistry is an open community resource for accessing chemical information. Nearly 500,000 chemical substances from CAS REGISTRY cover areas of community interest, including common and frequently regulated chemicals, and those relevant to high school and undergraduate chemistry classes. This chemical information, curated by our expert scientists, is provided in alignment with our mission as a division of the American Chemical Society. | |
Explanation | The data from CAS Common Chemistry is provided under a CC-BY-NC 4.0 license, unless otherwise stated. | |
Record name | Iron hydroxide oxide (Fe(OH)O) | |
Source | CAS Common Chemistry | |
URL | https://commonchemistry.cas.org/detail?cas_rn=20344-49-4 | |
Description | CAS Common Chemistry is an open community resource for accessing chemical information. Nearly 500,000 chemical substances from CAS REGISTRY cover areas of community interest, including common and frequently regulated chemicals, and those relevant to high school and undergraduate chemistry classes. This chemical information, curated by our expert scientists, is provided in alignment with our mission as a division of the American Chemical Society. | |
Explanation | The data from CAS Common Chemistry is provided under a CC-BY-NC 4.0 license, unless otherwise stated. | |
Record name | Goethite | |
Source | ChemIDplus | |
URL | https://pubchem.ncbi.nlm.nih.gov/substance/?source=chemidplus&sourceid=0001310141 | |
Description | ChemIDplus is a free, web search system that provides access to the structure and nomenclature authority files used for the identification of chemical substances cited in National Library of Medicine (NLM) databases, including the TOXNET system. | |
Record name | Lepidocrocite | |
Source | ChemIDplus | |
URL | https://pubchem.ncbi.nlm.nih.gov/substance/?source=chemidplus&sourceid=0012022376 | |
Description | ChemIDplus is a free, web search system that provides access to the structure and nomenclature authority files used for the identification of chemical substances cited in National Library of Medicine (NLM) databases, including the TOXNET system. | |
Record name | Hydrated ferric oxide | |
Source | ChemIDplus | |
URL | https://pubchem.ncbi.nlm.nih.gov/substance/?source=chemidplus&sourceid=0020344494 | |
Description | ChemIDplus is a free, web search system that provides access to the structure and nomenclature authority files used for the identification of chemical substances cited in National Library of Medicine (NLM) databases, including the TOXNET system. | |
Record name | Goethite (Fe(OH)O) | |
Source | EPA Chemicals under the TSCA | |
URL | https://www.epa.gov/chemicals-under-tsca | |
Description | EPA Chemicals under the Toxic Substances Control Act (TSCA) collection contains information on chemicals and their regulations under TSCA, including non-confidential content from the TSCA Chemical Substance Inventory and Chemical Data Reporting. | |
Record name | Akaganeite | |
Source | EPA DSSTox | |
URL | https://comptox.epa.gov/dashboard/DTXSID801318339 | |
Description | DSSTox provides a high quality public chemistry resource for supporting improved predictive toxicology. | |
Record name | Goethite (Fe(OH)O) | |
Source | European Chemicals Agency (ECHA) | |
URL | https://echa.europa.eu/substance-information/-/substanceinfo/100.013.797 | |
Description | The European Chemicals Agency (ECHA) is an agency of the European Union which is the driving force among regulatory authorities in implementing the EU's groundbreaking chemicals legislation for the benefit of human health and the environment as well as for innovation and competitiveness. | |
Explanation | Use of the information, documents and data from the ECHA website is subject to the terms and conditions of this Legal Notice, and subject to other binding limitations provided for under applicable law, the information, documents and data made available on the ECHA website may be reproduced, distributed and/or used, totally or in part, for non-commercial purposes provided that ECHA is acknowledged as the source: "Source: European Chemicals Agency, http://echa.europa.eu/". Such acknowledgement must be included in each copy of the material. ECHA permits and encourages organisations and individuals to create links to the ECHA website under the following cumulative conditions: Links can only be made to webpages that provide a link to the Legal Notice page. | |
Q1: What is the molecular formula and weight of goethite?
A1: Goethite's molecular formula is α-FeOOH. Its molecular weight is 88.85 g/mol.
Q2: How can spectroscopic techniques be used to characterize goethite?
A2: Various spectroscopic techniques are valuable for characterizing goethite:
- X-ray Diffraction (XRD): XRD reveals the crystalline structure and allows the identification and quantification of goethite in mixtures with other minerals. [, , ]
- Mössbauer Spectroscopy: This technique provides insights into the oxidation state of iron, the presence of impurities like aluminum, and the magnetic properties of goethite. [, , ]
- Infrared Spectroscopy (FTIR): FTIR helps identify specific functional groups present in goethite, such as hydroxyl groups and those arising from adsorbed species like carbonates or phosphates. [, ]
- X-ray Absorption Spectroscopy (XAS): XAS, including XANES and EXAFS, probes the local atomic environment around iron atoms in goethite, providing information on bond lengths, coordination numbers, and the presence of substituents. [, , ]
Q3: What is aluminum substitution in goethite and how does it impact its properties?
A3: Aluminum substitution in goethite refers to the replacement of some iron (Fe3+) ions in the goethite structure by aluminum (Al3+) ions. This substitution is common in natural goethites and can significantly influence its structural stability, surface charge, and reactivity, impacting its ability to adsorb pollutants and its dissolution behavior. [, , ]
Q4: How does goethite interact with organic matter in soils?
A4: Goethite interacts strongly with organic matter in soils through various mechanisms, including surface complexation, ligand exchange, and electrostatic interactions. These interactions can influence the fate and transport of organic pollutants in the environment and affect the bioavailability of nutrients. [, , ]
Q5: Can goethite act as a catalyst in environmental remediation?
A5: Yes, goethite has shown potential in environmental remediation due to its catalytic properties. For example, goethite can enhance the oxidation of organic pollutants like phenol in the presence of hydrogen peroxide. [] It can also facilitate the reduction of toxic Cr(VI) to less toxic Cr(III) in the presence of organic reductants. []
Q6: Does the presence of other minerals influence goethite's catalytic activity?
A6: Yes, the presence of other minerals can significantly impact goethite's catalytic activity. For instance, coexisting goethite can promote the transformation of ferrihydrite to goethite in the presence of Fe(II), influencing the fate of iron in redox-active environments. []
Q7: How is computational chemistry used to study goethite?
A7: Computational chemistry, particularly Density Functional Theory (DFT) calculations, has been employed to study the adsorption mechanisms of various species on goethite surfaces, providing insights into the geometry, charge distribution, and bonding characteristics of surface complexes. This information is valuable for understanding and predicting the fate and transport of pollutants in the environment. [, ]
Q8: How do structural modifications of goethite, like metal substitution, influence its reactivity?
A8: Structural modifications, particularly the incorporation of other metals like aluminum (Al) or tin (Sn), can significantly alter the reactivity of goethite. For instance, Sn-substituted goethites have demonstrated enhanced adsorption capacity for As(V) compared to pure goethite, highlighting their potential in arsenic removal technologies. []
Q9: How can the stability of goethite be enhanced for specific applications?
A9: The stability of goethite can be enhanced through various strategies. For example, incorporating goethite into composites with other materials like magnetic nanoparticles can improve its separation and recovery in water treatment applications. []
Q10: What are the environmental implications of goethite in soils?
A10: Goethite plays a crucial role in controlling the fate and transport of pollutants and nutrients in soils. Its ability to adsorb heavy metals, oxyanions like phosphate and arsenate, and organic pollutants makes it essential for maintaining soil and water quality. [, , , ]
Q11: How do different acids affect the dissolution of goethite?
A11: The dissolution of goethite is influenced by the type of acid present. Hydrochloric acid (HCl) typically dissolves goethite faster than perchloric acid (HClO4), suggesting that chloride ions may play a role in enhancing dissolution by forming surface complexes with iron. []
Q12: What factors can influence the dissolution rate of goethite?
A12: Several factors can influence the dissolution rate of goethite, including:
- pH: Dissolution is generally faster under acidic conditions. []
- Temperature: Higher temperatures generally increase dissolution rates. []
- Presence of ligands: Organic ligands can promote goethite dissolution by complexing with dissolved iron. []
- Presence of reductants: Reducing agents can enhance dissolution by converting Fe(III) in goethite to more soluble Fe(II). []
Disclaimer and Information on In-Vitro Research Products
Please be aware that all articles and product information presented on BenchChem are intended solely for informational purposes. The products available for purchase on BenchChem are specifically designed for in-vitro studies, which are conducted outside of living organisms. In-vitro studies, derived from the Latin term "in glass," involve experiments performed in controlled laboratory settings using cells or tissues. It is important to note that these products are not categorized as medicines or drugs, and they have not received approval from the FDA for the prevention, treatment, or cure of any medical condition, ailment, or disease. We must emphasize that any form of bodily introduction of these products into humans or animals is strictly prohibited by law. It is essential to adhere to these guidelines to ensure compliance with legal and ethical standards in research and experimentation.