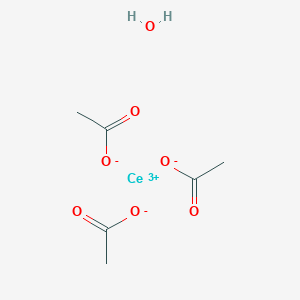
cerium (III) acetate monohydrate
Overview
Description
Chemical and Physical Properties Cerium(III) acetate monohydrate (Ce(CH₃COO)₃·H₂O) is a hydrated cerium salt with the molecular formula C₆H₁₁CeO₇ and a molecular weight of 317.25 g/mol . It is a white, crystalline powder that decomposes at 308°C, forming intermediates such as Ce₂O(CH₃COO)₄ and ultimately cerium(IV) oxide (CeO₂) upon calcination . The compound is soluble in water and has been historically utilized in topical burn treatments due to its bacteriostatic properties .
Synthesis and Applications The compound is synthesized via thermal decomposition of hydrated cerium acetate precursors. It serves as a critical precursor for CeO₂ nanoparticle (NP) synthesis, particularly in sol-gel methods and nanocomposite fabrication (e.g., ZnO/CeO₂) . Industrial applications span metallurgy, glass polishing, catalysts, and phosphors .
Preparation Methods
Synthetic Routes and Reaction Conditions: Cerium (III) acetate monohydrate can be synthesized by reacting cerium (III) carbonate with 50% acetic acid in an aqueous solution. The reaction proceeds as follows:
Ce2(CO3)3+6CH3COOH→2Ce(CH3COO)3+3H2O+3CO2↑
This reaction yields cerium (III) acetate along with water and carbon dioxide as by-products .
Industrial Production Methods: In industrial settings, this compound is produced using similar methods but on a larger scale. The process involves careful control of reaction conditions to ensure high purity and yield of the final product. The compound is then purified and crystallized to obtain the monohydrate form.
Chemical Reactions Analysis
Thermal Decomposition Pathway
Thermal analysis reveals a multi-stage decomposition process under inert or oxidative atmospheres:
In dry air, decomposition completes at 330°C , directly yielding CeO₂ . Under argon, intermediates such as Ce₈O₃(CH₃COO)₁₈ and Ce₂O₂CO₃ form .
Mechanistic Insights and Intermediate Products
The decomposition mechanism involves sequential ligand dissociation and oxidation:
-
Dehydration : Loss of 1.5 hydration water molecules at 133°C .
-
Crystallization : Exothermic transition to crystalline Ce(CH₃COO)₃ at 212°C .
-
Oxyacetate Formation : Endothermic decomposition at 286°C produces Ce₂O(CH₃COO)₄ .
-
Carbonate Intermediate : Ce₂O₂CO₃ forms above 350°C, decomposing to CeO₂ at 700°C .
Influence of Atmosphere on Decomposition
The reaction pathway varies significantly with atmospheric conditions:
Kinetics and Activation Energy
-
Activation energy for the crystallization of amorphous Ce(CH₃COO)₃ is 244 kJ/mol (Kissinger method) .
-
Heating rates impact decomposition dynamics, with slower rates (0.5°C/min) improving intermediate separation .
Structural and Analytical Characterization
-
TG-DTA-MS : Tracks mass loss (7.1–14.5%) and gas evolution (CO₂, acetone) .
-
XRD-DSC : Confirms phase transitions, including amorphous → crystalline Ce(CH₃COO)₃ .
Catalytic and Redox Behavior
Scientific Research Applications
Catalysis
Cerium (III) acetate monohydrate is recognized for its catalytic properties in organic reactions. It enhances reaction rates and yields, making it valuable in synthesizing fine chemicals, pharmaceuticals, and dyes.
- Case Study: In a study on the liquid-phase auto-oxidation of cresols, cerium (III) acetate was utilized as a catalyst, demonstrating its effectiveness in promoting oxidation reactions .
Catalytic Application | Reaction Type | Outcome |
---|---|---|
Cresol oxidation | Liquid-phase auto-oxidation | Increased yield of products |
Organic synthesis | Various transformations | Enhanced reaction rates |
Material Science
Cerium (III) acetate serves as a precursor for synthesizing cerium oxide nanoparticles (CeO₂), which are crucial in various advanced materials.
- Application in Electronics: Cerium oxide nanoparticles are used in fuel cells and solar cells due to their excellent catalytic properties and ability to store oxygen .
Material Science Application | End Product | Properties |
---|---|---|
Precursor for CeO₂ | Cerium oxide nanoparticles | High catalytic activity |
Thin films | High κ dielectrics | Improved electrical properties |
Environmental Remediation
Cerium (III) acetate plays a significant role in environmental applications, particularly in wastewater treatment. Its ability to degrade organic pollutants makes it an effective agent for remediation processes.
- Case Study: Research has shown that cerium (III) acetate can aid in the degradation of various organic contaminants in wastewater, highlighting its potential for environmental cleanup .
Environmental Application | Pollutant Type | Effectiveness |
---|---|---|
Wastewater treatment | Organic contaminants | Significant degradation rates |
Biomedical Applications
In biomedical research, cerium (III) acetate is explored for its antioxidant properties and potential use in drug delivery systems.
- Research Insight: Studies indicate that cerium ions can reduce oxidative stress in biological systems, suggesting applications in therapeutic contexts .
Biomedical Application | Mechanism of Action | Potential Use |
---|---|---|
Antioxidant activity | Reduces oxidative stress | Drug delivery systems |
Glass and Ceramics Industry
Cerium (III) acetate is widely used in the glass and ceramics industry to enhance durability and optical properties.
- Application Insight: When added to glass formulations, it improves resistance to scratches and thermal shock while also acting as a colorant that absorbs UV radiation .
Glass/Ceramics Application | Property Enhanced | Benefit |
---|---|---|
Glass manufacturing | Durability | Scratch resistance |
Optical glass | UV absorption | Improved stability |
Mechanism of Action
The mechanism by which cerium (III) acetate monohydrate exerts its effects is primarily through its ability to undergo redox reactions. Cerium can switch between its +3 and +4 oxidation states, allowing it to participate in various catalytic processes. This redox cycling is crucial for its antioxidant properties and its role in catalysis .
Comparison with Similar Compounds
Comparison with Similar Cerium(III) Compounds
Solubility and Chemical Properties
Cerium(III) acetate monohydrate exhibits moderate water solubility, contrasting with highly soluble cerium(III) nitrate hexahydrate (Ce(NO₃)₃·6H₂O) and cerium(III) chloride heptahydrate (CeCl₃·7H₂O). Insoluble compounds like cerium(III) phosphate (log Kₛₚ ~10⁻²⁹) highlight the variability in cerium salt solubility .
Table 1: Solubility and Stability of Cerium(III) Salts
Thermal Decomposition Pathways
- Cerium(III) acetate monohydrate: Decomposes stepwise between 300–700°C via intermediates (e.g., Ce₂O(CH₃COO)₄) to CeO₂ .
- Cerium(III) chloride heptahydrate : Requires higher temperatures (~400°C) for decomposition, risking chloride contamination in final products .
Reactivity in Chemical Reactions
- Acetate : Acts as a mild oxidant in redox reactions (e.g., silver-catalyzed oxidation of atropine) .
- Nitrate/Chloride: Preferred in radical cyclization and Grignard reactions due to higher ionic strength . Notably, cerium(III) chloride monohydrate reduces reaction yields compared to its heptahydrate form, emphasizing hydration state impacts .
Biological Activity
Cerium (III) acetate monohydrate, with the chemical formula and CAS number 206996-60-3, is a compound of cerium that has garnered attention for its diverse biological activities. This article reviews its biological properties, including its potential applications in medicine, environmental science, and materials science.
- Molecular Weight : 317.25 g/mol
- Appearance : White crystalline solid, soluble in water.
- IUPAC Name : Cerium(3+) triacetate.
1. Antioxidant Activity
Cerium (III) acetate has been studied for its antioxidant properties. It can scavenge free radicals, thereby reducing oxidative stress in biological systems. This property is particularly relevant in the context of diseases associated with oxidative damage, such as neurodegenerative disorders and cancer .
2. Antimicrobial Effects
Recent studies have highlighted the antimicrobial potential of cerium compounds, including cerium (III) acetate. Research indicates that cerium oxide nanoparticles (CeO₂ NPs), derived from cerium (III) acetate, exhibit significant antibacterial activity against pathogens like Porphyromonas gingivalis and Prevotella intermedia. The mechanism involves the generation of reactive oxygen species (ROS) upon contact with bacterial membranes, leading to cell death .
3. Osteogenic Differentiation
Cerium oxide nanoparticles have shown promise in promoting osteogenic differentiation in human periodontal ligament cells (hPDLCs). Studies demonstrated that these nanoparticles enhance alkaline phosphatase activity and mineralization, indicating their potential use in bone regeneration therapies .
Case Study 1: Antibacterial Activity
A study investigated the antibacterial effects of cerium oxide nanoparticles synthesized from cerium (III) acetate against anaerobic pathogens. The results indicated a significant reduction in bacterial viability, suggesting that cerium compounds could be utilized in clinical settings to manage infections .
Pathogen | Minimum Inhibitory Concentration (MIC) |
---|---|
Porphyromonas gingivalis | 0.5 mg/mL |
Prevotella intermedia | 0.8 mg/mL |
Case Study 2: Oxidative Stress Reduction
In another study focused on oxidative stress, researchers treated hPDLCs with hydrogen peroxide to induce stress and then exposed them to cerium oxide nanoparticles. The findings revealed that the nanoparticles significantly improved cell viability and reduced ROS levels, demonstrating their potential as therapeutic agents in oxidative stress-related conditions .
The biological activity of cerium (III) acetate is largely attributed to its ability to modulate redox reactions and generate ROS under specific conditions. The reversible oxidation state of cerium ions (Ce³⁺ to Ce⁴⁺) plays a crucial role in these processes:
- Antioxidant Mechanism : Cerium ions can act as electron donors or acceptors, facilitating redox reactions that mitigate oxidative damage.
- Antimicrobial Mechanism : The interaction of cerium oxide with bacterial membranes leads to ROS generation, which disrupts cellular functions and induces apoptosis in bacteria .
Applications in Material Science
Cerium (III) acetate is not only relevant for its biological activities but also finds applications in material science:
- Catalysis : It serves as an effective catalyst for various organic reactions, enhancing yields and reaction rates .
- Nanoparticle Synthesis : Used as a precursor for cerium oxide nanoparticles, which have applications in catalysis, electronics, and environmental remediation .
- Glass and Ceramics : Enhances the durability and optical properties of glass products .
Q & A
Basic Research Questions
Q. What are the standard synthesis protocols for cerium(III) acetate monohydrate, and what safety precautions are critical during handling?
Methodological Answer: Cerium(III) acetate monohydrate is typically synthesized via controlled precipitation or solvothermal methods. For example, in nanocrystal synthesis, Schlenk techniques under an argon atmosphere are employed to prevent oxidation and ensure reagent purity . Key precautions include:
- Inert Atmosphere: Use of argon/nitrogen gloveboxes to minimize exposure to moisture and oxygen.
- Waste Management: Segregation of waste for professional disposal to avoid environmental contamination (e.g., cerium-containing residues require specialized treatment) .
- Personal Protective Equipment (PPE): Tightly sealed goggles and gloves to prevent ocular/skin contact, as the compound is a mild irritant .
Q. Which analytical techniques are most effective for characterizing cerium(III) acetate monohydrate’s purity and structural properties?
Methodological Answer:
- X-Ray Diffraction (XRD): Confirms crystallinity and phase purity by comparing peaks to reference databases (e.g., used to verify (NH₄)₂Ce(PO₄)₂·H₂O in catalyst studies) .
- Thermogravimetric Analysis (TGA): Determines hydration state and thermal stability by measuring mass loss at elevated temperatures .
- Inductively Coupled Plasma Mass Spectrometry (ICP-MS): Quantifies trace metal impurities (e.g., ≤150 ppm rare earth contaminants) .
Advanced Research Questions
Q. How do reaction parameters (temperature, precursor ratios) influence the morphology of CeO₂ nanostructures derived from cerium(III) acetate monohydrate?
Methodological Answer:
- Temperature Control: Higher temperatures (e.g., 700°C in argon) during electrospinning promote the formation of Co/CeO₂-doped carbon nanofibers with enhanced surface area and conductivity .
- Precursor Ratios: Adjusting the molar ratio of cerium acetate to cobalt acetate (e.g., 75:25 wt%) tailors pore structure and catalytic activity in supercapacitor electrodes .
- Doping Effects: Co-doping with Fe/Ni alters oxygen vacancy content in CeO₂, improving electrocatalytic performance in water-splitting reactions .
Q. What experimental strategies can resolve contradictions in catalytic activity data for cerium(III) acetate monohydrate-based catalysts?
Methodological Answer:
- Systematic Variable Testing: Use uniform experimental design to isolate factors like reaction time, acid/alcohol molar ratios, and catalyst loading (e.g., in benzyl acetate synthesis) .
- Surface Analysis: Employ X-ray photoelectron spectroscopy (XPS) to correlate surface Ce³⁺/Ce⁴⁺ ratios with catalytic efficiency .
- Cross-Validation: Compare kinetic data across studies using standardized conditions (e.g., inert atmosphere, controlled humidity) to minimize discrepancies .
Q. How does cerium(III) acetate monohydrate perform as a dopant in quantum dots, and what are the challenges in achieving uniform energy transfer?
Methodological Answer:
- Synthesis Optimization: Co-doping with Eu³⁺ or Tb³⁺ in ZnS quantum dots requires precise stoichiometry (e.g., 1–5 mol%) to balance luminescence intensity and quenching effects .
- Surface Passivation: Use oleic acid and 1-octadecene to stabilize nanocrystals and reduce defect-related energy loss .
- Characterization Challenges: Time-resolved photoluminescence (TRPL) and TEM are critical to assess dopant distribution and energy transfer efficiency .
Q. What are the best practices for integrating cerium(III) acetate monohydrate into electrochemical systems (e.g., supercapacitors, fuel cells)?
Methodological Answer:
- Electrode Fabrication: Electrospinning cerium acetate with poly(vinyl alcohol) and cobalt acetate produces carbon nanofibers with high capacitance (~450 F/g at 1 A/g) .
- Doping Protocols: Co-doping with transition metals (Ni, Fe) enhances oxygen evolution reaction (OER) activity by modulating CeO₂’s redox properties .
- Stability Testing: Long-term cyclic voltammetry (e.g., 10,000 cycles) under acidic/alkaline conditions evaluates structural degradation .
Q. Data Contradiction Analysis
Q. Why do studies report varying optical properties for Ce³⁺-doped nanomaterials, and how can these inconsistencies be addressed?
Methodological Answer:
- Synthesis Variability: Differences in precursor purity (e.g., 99.9% vs. 99.99% metals basis) and hydration states significantly alter emission spectra .
- Measurement Techniques: Standardize excitation wavelengths and solvent systems (e.g., toluene vs. aqueous media) to ensure comparability .
- Sample Preparation: Control nanoparticle size distribution via centrifugation or size-selective precipitation to minimize scattering effects .
Properties
IUPAC Name |
cerium(3+);triacetate;hydrate | |
---|---|---|
Details | Computed by LexiChem 2.6.6 (PubChem release 2019.06.18) | |
Source | PubChem | |
URL | https://pubchem.ncbi.nlm.nih.gov | |
Description | Data deposited in or computed by PubChem | |
InChI |
InChI=1S/3C2H4O2.Ce.H2O/c3*1-2(3)4;;/h3*1H3,(H,3,4);;1H2/q;;;+3;/p-3 | |
Details | Computed by InChI 1.0.5 (PubChem release 2019.06.18) | |
Source | PubChem | |
URL | https://pubchem.ncbi.nlm.nih.gov | |
Description | Data deposited in or computed by PubChem | |
InChI Key |
AERUOEZHIAYQQL-UHFFFAOYSA-K | |
Details | Computed by InChI 1.0.5 (PubChem release 2019.06.18) | |
Source | PubChem | |
URL | https://pubchem.ncbi.nlm.nih.gov | |
Description | Data deposited in or computed by PubChem | |
Canonical SMILES |
CC(=O)[O-].CC(=O)[O-].CC(=O)[O-].O.[Ce+3] | |
Details | Computed by OEChem 2.1.5 (PubChem release 2019.06.18) | |
Source | PubChem | |
URL | https://pubchem.ncbi.nlm.nih.gov | |
Description | Data deposited in or computed by PubChem | |
Molecular Formula |
C6H11CeO7 | |
Details | Computed by PubChem 2.1 (PubChem release 2019.06.18) | |
Source | PubChem | |
URL | https://pubchem.ncbi.nlm.nih.gov | |
Description | Data deposited in or computed by PubChem | |
Molecular Weight |
335.26 g/mol | |
Details | Computed by PubChem 2.1 (PubChem release 2021.05.07) | |
Source | PubChem | |
URL | https://pubchem.ncbi.nlm.nih.gov | |
Description | Data deposited in or computed by PubChem | |
Retrosynthesis Analysis
AI-Powered Synthesis Planning: Our tool employs the Template_relevance Pistachio, Template_relevance Bkms_metabolic, Template_relevance Pistachio_ringbreaker, Template_relevance Reaxys, Template_relevance Reaxys_biocatalysis model, leveraging a vast database of chemical reactions to predict feasible synthetic routes.
One-Step Synthesis Focus: Specifically designed for one-step synthesis, it provides concise and direct routes for your target compounds, streamlining the synthesis process.
Accurate Predictions: Utilizing the extensive PISTACHIO, BKMS_METABOLIC, PISTACHIO_RINGBREAKER, REAXYS, REAXYS_BIOCATALYSIS database, our tool offers high-accuracy predictions, reflecting the latest in chemical research and data.
Strategy Settings
Precursor scoring | Relevance Heuristic |
---|---|
Min. plausibility | 0.01 |
Model | Template_relevance |
Template Set | Pistachio/Bkms_metabolic/Pistachio_ringbreaker/Reaxys/Reaxys_biocatalysis |
Top-N result to add to graph | 6 |
Feasible Synthetic Routes
Disclaimer and Information on In-Vitro Research Products
Please be aware that all articles and product information presented on BenchChem are intended solely for informational purposes. The products available for purchase on BenchChem are specifically designed for in-vitro studies, which are conducted outside of living organisms. In-vitro studies, derived from the Latin term "in glass," involve experiments performed in controlled laboratory settings using cells or tissues. It is important to note that these products are not categorized as medicines or drugs, and they have not received approval from the FDA for the prevention, treatment, or cure of any medical condition, ailment, or disease. We must emphasize that any form of bodily introduction of these products into humans or animals is strictly prohibited by law. It is essential to adhere to these guidelines to ensure compliance with legal and ethical standards in research and experimentation.