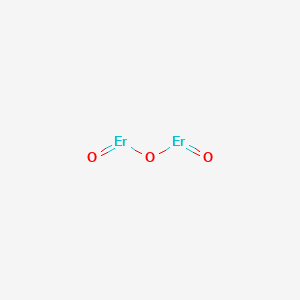
Erbium oxide
Overview
Description
Erbium oxide, also known as erbia, is an inorganic compound with the formula Er₂O₃. It is a pink paramagnetic solid that finds uses in various optical materials. This compound is notable for its ability to upconvert photons, converting infrared or visible radiation into ultraviolet or violet radiation through multiple energy transfers or absorptions .
Mechanism of Action
Target of Action
Erbium oxide, also known as Erbium(III) oxide, is an inorganic compound with the formula Er2O3 . The primary targets of this compound are the Er3+ centers, which are octahedral . These centers play a crucial role in the compound’s various optical properties .
Mode of Action
This compound interacts with its targets, the Er3+ centers, through a process known as photon upconversion . This process involves the conversion of infrared or visible radiation (low energy light) to ultraviolet or violet radiation (higher energy light) via multiple transfer or absorption of energy . This unique interaction results in the compound’s photoluminescence properties .
Biochemical Pathways
The compound’s ability to upconvert photons suggests that it may influence pathways related to light absorption and energy transfer .
Pharmacokinetics
It is known that this compound is insoluble in water and soluble in mineral acids . This solubility profile may influence the compound’s bioavailability.
Result of Action
The primary result of this compound’s action is its ability to upconvert photons . This property allows this compound to convert low energy light into higher energy light, a feature that is utilized in various optical materials . Additionally, this compound nanoparticles possess photoluminescence properties .
Biochemical Analysis
Biochemical Properties
Erbium oxide nanoparticles have been synthesized and studied for their biochemical properties . They have been found to interact with enzymes such as α-glucosidase and α-amylase . A dose-dependent inhibition was observed against these enzymes, indicating a potential role of this compound in biochemical reactions .
Cellular Effects
This compound nanoparticles have shown to have effects on cells, particularly cancer cells . Exposure to these nanoparticles for 72 hours inhibited growth and caused death of Hep-G2 cells in a concentration-dependent manner . It was also found to cause high DNA damage and extra-production of intracellular reactive oxygen species (ROS) in Hep-G2 cells .
Molecular Mechanism
The molecular mechanism of action of this compound is related to its ability to generate reactive oxygen species (ROS). The extra ROS generation causes high DNA damage induction and alterations of apoptotic genes . This suggests that this compound exerts its effects at the molecular level through binding interactions with biomolecules, potentially leading to enzyme inhibition or activation, and changes in gene expression .
Temporal Effects in Laboratory Settings
It has been observed that this compound nanoparticles can cause time- and concentration-dependent cytotoxicity toward normal human skin fibroblast (HSF) cells .
Dosage Effects in Animal Models
In animal models, this compound nanoparticles have shown antidiabetic potential . A dose-dependent improvement in glycemic control was observed in diabetic animals treated with these nanoparticles .
Metabolic Pathways
Given its interactions with enzymes such as α-glucosidase and α-amylase, it is likely that it may play a role in carbohydrate metabolism .
Preparation Methods
Synthetic Routes and Reaction Conditions
Erbium oxide can be synthesized through several methods. One common method involves burning erbium metal in the presence of oxygen. The reaction is as follows: [ 4Er + 3O₂ → 2Er₂O₃ ]
Another method involves dissolving erbium salts, such as erbium chloride or erbium nitrate, in a solvent and then adding an oxidizing agent like hydrogen peroxide or oxygen. The solution is then allowed to react at an appropriate temperature to produce this compound precipitate .
Industrial Production Methods
In industrial settings, this compound is typically produced by dissolving erbium salts in a solvent to form a homogeneous solution. An oxidizing agent is then added slowly while stirring to ensure thorough mixing. The solution is allowed to react at a controlled temperature to produce this compound precipitate, which is then filtered, washed with deionized water, and dried to obtain the final product .
Chemical Reactions Analysis
Types of Reactions
Erbium oxide undergoes several types of chemical reactions, including:
Oxidation: Erbium metal reacts with oxygen to form this compound.
Reduction: this compound can be reduced to erbium metal using strong reducing agents.
Acid-Base Reactions: this compound reacts with acids to form erbium salts.
Common Reagents and Conditions
Oxidation: Oxygen or air at high temperatures.
Reduction: Strong reducing agents like hydrogen or carbon at elevated temperatures.
Acid-Base Reactions: Mineral acids such as hydrochloric acid.
Major Products Formed
Oxidation: this compound (Er₂O₃).
Reduction: Erbium metal (Er).
Acid-Base Reactions: Erbium salts, such as erbium chloride (ErCl₃) and water (H₂O).
Scientific Research Applications
Erbium oxide has a wide range of scientific research applications, including:
Optical Materials: Used in the production of optical fibers and amplifiers due to its ability to upconvert photons.
Nanomedicine: This compound nanoparticles have been studied for their potential in drug delivery and bioimaging.
Supercapacitors: Used as electrodes in supercapacitors due to its excellent electrochemical properties.
Wastewater Treatment: This compound-doped materials are used in the photocatalytic degradation of pollutants.
Comparison with Similar Compounds
Erbium oxide can be compared with other rare earth oxides such as:
Holmium oxide (Ho₂O₃): Similar in structure and properties but has different photoluminescent characteristics.
Thulium oxide (Tm₂O₃): Also used in optical materials but has different upconversion properties.
Yttthis compound (Yb₂O₃): Used in similar applications but has different electronic and optical properties.
This compound is unique due to its specific photoluminescent properties and its ability to upconvert photons, making it particularly valuable in optical and photonic applications.
Properties
IUPAC Name |
oxo(oxoerbiooxy)erbium | |
---|---|---|
Details | Computed by Lexichem TK 2.7.0 (PubChem release 2021.05.07) | |
Source | PubChem | |
URL | https://pubchem.ncbi.nlm.nih.gov | |
Description | Data deposited in or computed by PubChem | |
InChI |
InChI=1S/2Er.3O | |
Details | Computed by InChI 1.0.6 (PubChem release 2021.05.07) | |
Source | PubChem | |
URL | https://pubchem.ncbi.nlm.nih.gov | |
Description | Data deposited in or computed by PubChem | |
InChI Key |
VQCBHWLJZDBHOS-UHFFFAOYSA-N | |
Details | Computed by InChI 1.0.6 (PubChem release 2021.05.07) | |
Source | PubChem | |
URL | https://pubchem.ncbi.nlm.nih.gov | |
Description | Data deposited in or computed by PubChem | |
Canonical SMILES |
O=[Er]O[Er]=O | |
Details | Computed by OEChem 2.3.0 (PubChem release 2021.05.07) | |
Source | PubChem | |
URL | https://pubchem.ncbi.nlm.nih.gov | |
Description | Data deposited in or computed by PubChem | |
Molecular Formula |
Er2O3 | |
Details | Computed by PubChem 2.1 (PubChem release 2021.05.07) | |
Record name | Erbium(III) oxide | |
Source | Wikipedia | |
URL | https://en.wikipedia.org/wiki/Erbium(III)_oxide | |
Description | Chemical information link to Wikipedia. | |
Details | Computed by PubChem 2.1 (PubChem release 2021.05.07) | |
Source | PubChem | |
URL | https://pubchem.ncbi.nlm.nih.gov | |
Description | Data deposited in or computed by PubChem | |
Molecular Weight |
382.52 g/mol | |
Details | Computed by PubChem 2.1 (PubChem release 2021.05.07) | |
Source | PubChem | |
URL | https://pubchem.ncbi.nlm.nih.gov | |
Description | Data deposited in or computed by PubChem | |
Physical Description |
Water insoluble, pink powder; [Hawley] | |
Record name | Erbium oxide | |
Source | Haz-Map, Information on Hazardous Chemicals and Occupational Diseases | |
URL | https://haz-map.com/Agents/2102 | |
Description | Haz-Map® is an occupational health database designed for health and safety professionals and for consumers seeking information about the adverse effects of workplace exposures to chemical and biological agents. | |
Explanation | Copyright (c) 2022 Haz-Map(R). All rights reserved. Unless otherwise indicated, all materials from Haz-Map are copyrighted by Haz-Map(R). No part of these materials, either text or image may be used for any purpose other than for personal use. Therefore, reproduction, modification, storage in a retrieval system or retransmission, in any form or by any means, electronic, mechanical or otherwise, for reasons other than personal use, is strictly prohibited without prior written permission. | |
Q1: What is the molecular formula and weight of erbium oxide?
A1: The molecular formula of this compound is Er₂O₃. Its molecular weight is 382.52 g/mol.
Q2: What is unique about the spectral emission of this compound?
A2: this compound exhibits selective line emission, meaning it has a high emittance at specific wavelengths and low emittance in the rest of the infrared spectrum. [, , , ] This makes it suitable for applications like thermophotovoltaic generators (TPV), as its emission can be tailored to match the response of specific photovoltaic cells, such as InGaAs cells. [, , ]
Q3: Does this compound change its optical properties with temperature?
A4: Yes, experimental measurements show that the emissivity of this compound is temperature-dependent. [] While the emissivity remains low and constant within the 2 to 5-micron wavelength range, its behavior at other wavelengths might vary with temperature. []
Q4: How does the presence of this compound impact the structure of tellurite-based glasses?
A5: The addition of this compound to tellurite-based glasses affects the glass network by increasing the number of non-bridging oxygens. [] This influences the bonding structure of components like TeO2, ZnO, and B2O3 within the glass matrix. []
Q5: What is the thermal shock resistance of pure this compound cylindrical structures?
A6: Pure this compound cylinders exhibit poor thermal shock resistance, making them prone to cracking and resulting in a short thermal cycle life. [] This limits their use as radiating mantles in high-temperature applications like thermophotovoltaic converter systems. []
Q6: Can this compound be used as an antireflective coating for silicon solar cells?
A8: Yes, this compound thin films, when subjected to specific rapid thermal annealing treatments, exhibit good antireflective properties on silicon. [] Annealing at 500 °C with controlled ramp and cooling rates reduces the average reflection significantly, leading to a substantial increase in the short circuit current of silicon-based photovoltaic devices. []
Q7: Is this compound compatible with liquid lithium, and what are its potential applications in fusion reactors?
A9: this compound exhibits good chemical compatibility with liquid lithium and is considered a promising candidate for magnetohydrodynamics (MHD) coatings in fusion reactors. [] These coatings, typically applied to the inside of tubing, help reduce pressure drops caused by liquid lithium flowing through strong magnetic fields. []
Q8: How does the substrate temperature affect the properties of this compound coatings deposited by sputtering?
A10: The substrate temperature during pulsed magnetron sputtering significantly influences the crystallization and properties of this compound coatings. [] High deposition rates and low substrate temperatures typically lead to the formation of a monoclinic Er₂O₃ phase. [] Increasing the substrate temperature affects the coating's crystalline quality and influences its electrical resistivity, impacting its suitability for applications like insulating coatings in fusion reactors. []
Q9: What are some applications of this compound in biomedical assays?
A11: this compound nanoparticles, alone or in composites with other materials like iron oxide, are being explored for their potential in biomedical assays. [] These applications stem from the material's unique optical and magnetic properties, making it suitable for techniques requiring fluorescence detection or magnetic manipulation. []
Q10: How can this compound be used in optical dosimetry for ionizing radiation fields?
A12: this compound exhibits radioluminescence in the infrared region, making it suitable for optical dosimetry in ionizing radiation fields. [] When used with radiation-resistant optical fibers, it offers advantages over conventional electric dosimetry systems, especially in environments with high electromagnetic noise like nuclear fusion systems. []
Q11: How is this compound used in the development of up-conversion phosphors?
A13: this compound is a key component in up-conversion phosphors, where it acts as an activator ion responsible for the up-conversion process. [, ] When combined with other materials like TiO2 and ZnO using methods like the MOD method, it allows for efficient energy transfer and controllable emission characteristics. [, ] These phosphors have potential applications in displays, solid-state lighting, and scintillator technology. [, ]
Q12: Can this compound be used as a gate dielectric in MOS technology?
A14: Yes, this compound shows promise as a potential gate dielectric in MOS technology due to its dielectric properties and compatibility with silicon. [] Research suggests that the presence of an interfacial SiO2 layer during deposition and subsequent annealing processes can significantly influence the effective dielectric constant and leakage current of this compound films. [] This control over electrical properties makes it attractive for developing next-generation transistors. []
Q13: What is the role of this compound in the solar thermochemical conversion of CO2?
A15: this compound is explored as a redox material in solar thermochemical cycles for splitting CO2. [] The process involves a cyclic reduction and oxidation of this compound at high temperatures driven by concentrated solar energy. [] While promising, the high temperatures required for efficient operation currently pose a challenge for wider implementation. []
Q14: How is this compound utilized in the preparation of rare-earth erbium alloys?
A16: this compound, alongside other rare-earth oxides, serves as the precursor material for producing rare-earth erbium alloys via electrolysis in a fluoride molten salt system. [] This method offers a relatively simple and environmentally friendly approach compared to conventional techniques, with the added benefit of producing minimal pollutants. []
Q15: Are there any computational studies on helium trapping in erbium hydrides?
A17: Yes, density functional theory (DFT) calculations have been employed to investigate the interaction of helium with this compound precipitates in erbium hydrides. [] These calculations provide insights into the energetic favorability of helium residing in the oxide phase compared to the bulk hydride, suggesting a possible mechanism for helium trapping during the aging of erbium tritides. []
Q16: What analytical techniques are commonly used to characterize this compound and related materials?
A16: Various analytical methods are employed to characterize this compound and related materials. These include:
- X-ray diffraction (XRD): for determining crystal structure and phase identification [, , , , , , ].
- Transmission electron microscopy (TEM): for visualizing nanoscale morphology and structure [, , ].
- Atomic force microscopy (AFM): for studying surface morphology and roughness [, ].
- Raman spectroscopy: for analyzing vibrational modes and bonding information [, ].
- X-ray photoelectron spectroscopy (XPS): for determining elemental composition and chemical states [].
- Photoluminescence (PL) spectroscopy: for investigating optical properties and energy transfer processes [, , , ].
- UV-Vis spectroscopy: for studying electronic transitions and optical band gap [, , ].
- Fourier-transform infrared (FTIR) spectroscopy: for identifying functional groups and bonding characteristics [, , ].
- Inductively coupled plasma mass spectrometry (ICP-MS): for quantifying elemental impurities [].
- Auger electron spectroscopy (AES): for analyzing elemental composition and depth profiling [].
- Scanning electron microscopy (SEM): for examining surface morphology and microstructure [].
Disclaimer and Information on In-Vitro Research Products
Please be aware that all articles and product information presented on BenchChem are intended solely for informational purposes. The products available for purchase on BenchChem are specifically designed for in-vitro studies, which are conducted outside of living organisms. In-vitro studies, derived from the Latin term "in glass," involve experiments performed in controlled laboratory settings using cells or tissues. It is important to note that these products are not categorized as medicines or drugs, and they have not received approval from the FDA for the prevention, treatment, or cure of any medical condition, ailment, or disease. We must emphasize that any form of bodily introduction of these products into humans or animals is strictly prohibited by law. It is essential to adhere to these guidelines to ensure compliance with legal and ethical standards in research and experimentation.