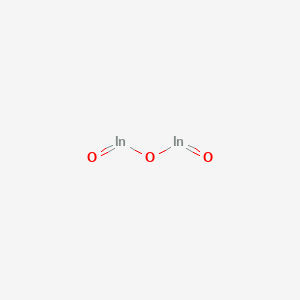
Indium oxide (In2O3)
- Click on QUICK INQUIRY to receive a quote from our team of experts.
- With the quality product at a COMPETITIVE price, you can focus more on your research.
Overview
Description
Indium oxide (In2O3) is a useful research compound. Its molecular formula is In2O3 and its molecular weight is 277.63 g/mol. The purity is usually 95%.
BenchChem offers high-quality Indium oxide (In2O3) suitable for many research applications. Different packaging options are available to accommodate customers' requirements. Please inquire for more information about Indium oxide (In2O3) including the price, delivery time, and more detailed information at info@benchchem.com.
Scientific Research Applications
Optoelectronic Applications : In2O3 is used in optoelectronic devices due to its transparent conducting properties. It is suitable for applications in flat-panel displays, photovoltaics, and light-emitting diodes (J. Elam et al., 2006).
Gas Sensors : In2O3 shows promise in gas sensor technology, particularly for detecting toxic gases like NH3 at room temperature. This is due to its high electrical conductivity and transparency (N. Du et al., 2007).
Photovoltaic Devices : Indium oxide thin films are important in photovoltaic applications. Their properties can be influenced by varying precursor concentrations, impacting their suitability for solar cell technology (L. N. Lau et al., 2015).
Toxicology and Safety : The toxicology of In2O3, especially in the context of occupational exposure, is a critical area of study. It has been found that In2O3 particles can accumulate in the lungs and cause severe effects (E. Bomhard, 2018).
Perovskite Solar Cells : In2O3 is utilized in perovskite solar cells as an electron selective layer, enhancing their efficiency. Its optical and electrical properties like high mobility and wide band gap make it suitable for these applications (Minchao Qin et al., 2016).
Surface Speciation and Oxidation : Understanding the speciation and oxidation of In2O3 surfaces is crucial for its use in electronics, particularly in electrodes and electrocatalytic applications (Z. Detweiler et al., 2016).
Nanostructure Synthesis for Optoelectronic Devices : Indium oxide nanostructures can be synthesized in various shapes, which affects their optoelectronic properties. This shape control is crucial for enhancing the performance of devices like photoelectrochemical cells (Dipak V. Shinde et al., 2014).
Thermal Atomic Layer Deposition (ALD) : ALD is used for depositing In2O3 thin films, which are significant in optoelectronic applications. The process yields films with high optical transmittance and low resistivity, suitable for microelectronic displays (Pamburayi Mpofu et al., 2021).
Photocatalysis : In2O3 shows activity for photocatalytic CO2 reduction, making it a potential candidate for environmental applications (Yun‐Xiang Pan et al., 2017).
Flexible Electronics : Indium oxide's ability to be deposited at low temperatures makes it suitable for flexible electronics, particularly in thin-film transistors and integrated circuits (L. Petti et al., 2015).
Mechanism of Action
Target of Action
Indium Oxide (In2O3) is primarily used in the field of semiconductors . It serves as a resistive element in integrated circuits . The primary targets of In2O3 are the electronic components where it is applied.
Pharmacokinetics
It’s worth noting that indium compounds have low solubility and are poorly absorbed in the body .
Action Environment
The action of Indium Oxide is influenced by environmental factors such as temperature and pressure. It is thermally stable , making it suitable for use in various environments.
properties
IUPAC Name |
oxo(oxoindiganyloxy)indigane |
Source
|
---|---|---|
Details | Computed by LexiChem 2.6.6 (PubChem release 2019.06.18) | |
Source | PubChem | |
URL | https://pubchem.ncbi.nlm.nih.gov | |
Description | Data deposited in or computed by PubChem | |
InChI |
InChI=1S/2In.3O |
Source
|
Details | Computed by InChI 1.0.5 (PubChem release 2019.06.18) | |
Source | PubChem | |
URL | https://pubchem.ncbi.nlm.nih.gov | |
Description | Data deposited in or computed by PubChem | |
InChI Key |
SHTGRZNPWBITMM-UHFFFAOYSA-N |
Source
|
Details | Computed by InChI 1.0.5 (PubChem release 2019.06.18) | |
Source | PubChem | |
URL | https://pubchem.ncbi.nlm.nih.gov | |
Description | Data deposited in or computed by PubChem | |
Canonical SMILES |
O=[In]O[In]=O |
Source
|
Details | Computed by OEChem 2.1.5 (PubChem release 2019.06.18) | |
Source | PubChem | |
URL | https://pubchem.ncbi.nlm.nih.gov | |
Description | Data deposited in or computed by PubChem | |
Molecular Formula |
In2O3 |
Source
|
Details | Computed by PubChem 2.1 (PubChem release 2019.06.18) | |
Source | PubChem | |
URL | https://pubchem.ncbi.nlm.nih.gov | |
Description | Data deposited in or computed by PubChem | |
Molecular Weight |
277.63 g/mol |
Source
|
Details | Computed by PubChem 2.1 (PubChem release 2021.05.07) | |
Source | PubChem | |
URL | https://pubchem.ncbi.nlm.nih.gov | |
Description | Data deposited in or computed by PubChem | |
Q & A
Q1: What is the molecular formula and weight of Indium Oxide?
A1: Indium oxide has the molecular formula In2O3 and a molecular weight of 277.64 g/mol.
Q2: What is the crystal structure of In2O3?
A2: In2O3 commonly exists in two crystal structures: * Cubic bixbyite structure (Ia3): This is the thermodynamically stable phase at ambient conditions. [, ] * Rhombohedral corundum structure (R3c): This phase is accessible under high pressure and temperature conditions. [, ]
Q3: What spectroscopic techniques are used to characterize In2O3?
A3: Researchers utilize various techniques, including:* X-ray diffraction (XRD): To determine the crystal structure and phase purity of In2O3. [, , , , , , ]* Scanning Electron Microscopy (SEM): To visualize the morphology and size of In2O3 particles. [, , , , , , , , ]* Transmission Electron Microscopy (TEM): To analyze the size, shape, and crystallinity of In2O3 nanostructures. []* Energy-dispersive X-ray spectroscopy (EDS/EDX): To determine the elemental composition and confirm the presence of indium and oxygen. [, , ] * X-ray photoelectron spectroscopy (XPS): To investigate the chemical states and surface composition of In2O3. [, ]* Raman spectroscopy: To study the vibrational modes and identify different phases of In2O3. [, ]* Fourier-transform infrared spectroscopy (FTIR): To analyze the chemical bonding and identify functional groups present in In2O3. [, , ]* UV-Vis Spectroscopy: To investigate the optical properties of In2O3 thin films, such as transmittance and bandgap energy. [, , , , , , ]
Q4: How does the performance of In2O3 change under different annealing conditions?
A4: Annealing temperature and atmosphere significantly influence In2O3 properties. Higher annealing temperatures generally improve crystallinity, leading to enhanced electrical conductivity. [, , ] Annealing in a reducing atmosphere can induce phase transitions and alter the electrical properties. []
Q5: What factors affect the stability of In2O3 thin films in electronic devices?
A5: In2O3 thin film transistors (TFTs) can suffer from performance degradation when exposed to ambient conditions, particularly oxygen and moisture. [] To improve stability, researchers explore passivation layers like aluminum oxide (Al2O3), which reduce off-current and enhance the on/off current ratio. []
Q6: What makes In2O3 suitable for flexible electronics?
A6: Solution-processed In2O3 can be deposited at low temperatures, making it compatible with flexible substrates like polyimide. [, ] Additionally, In2O3 thin films can withstand bending without significant performance degradation, making them suitable for flexible devices. [, ]
Q7: What are the photocatalytic properties of In2O3?
A7: In2O3 exhibits photocatalytic activity under UV light irradiation. [, ] This property makes In2O3 nanoparticles promising for applications such as degrading organic pollutants like crystal violet dye in wastewater treatment. []
Q8: How is In2O3 used in gas sensing applications?
A8: The electrical conductivity of In2O3 changes upon exposure to various gases. [, , , , ] This property enables its use in gas sensors. For instance, researchers developed In2O3-based sensors for detecting ethanol, NO2, and 1-butylamine. [, , , ] The sensitivity and selectivity of these sensors can be tuned by controlling the morphology, doping, and operating temperature. [, , , ]
Q9: How is computational chemistry used to understand In2O3 properties?
A9: Density Functional Theory (DFT) calculations help investigate the structural, electronic, and thermodynamic properties of various In2O3 polymorphs. [] These calculations can predict the stability of different phases, electronic band structures, and mechanical properties like elastic moduli. []
Q10: Can computational methods predict the performance of In2O3 in specific applications?
A10: Yes, computational modeling can help predict the performance of In2O3. For example, researchers used simulations to demonstrate enhanced sensitivity and figure-of-merit (FOM) in a plasmonic structure incorporating In2O3 for sensing applications in the visible and infrared regions. []
Q11: How do the properties of In2O3 change at the nanoscale?
A11: In2O3 nanostructures, such as nanowires, nanorods, and quantum dots, exhibit unique properties compared to their bulk counterparts. These include enhanced surface area, quantum confinement effects, and increased surface defect sites. [, , , , , , , , , , , ]
Q12: What are some specific applications of In2O3 nanostructures?
A12:* High-performance photoelectrochemical UV photodetectors: In2O3 nanocubes and microrods show promise for UV detection due to their high UV absorption and efficient charge transport properties. [, ]* Humidity sensors: In2O3 quantum dots with their large surface area and porous structure are highly sensitive to moisture, making them suitable for humidity sensing applications. []* Flexible synaptic transistors: Electrospun In2O3 nanofibers enable the fabrication of flexible synaptic transistors for neuromorphic computing applications. []
Q13: How does doping affect the properties of In2O3?
A13: Doping In2O3 with elements like tin (Sn), zinc (Zn), and europium (Eu) can significantly modify its electrical and optical properties. [, , , , , ] For example:
- Tin (Sn) doping: Improves electrical conductivity by increasing carrier concentration, making it suitable for transparent conductive oxide (TCO) applications. [, , ]
- Zinc (Zn) doping: Enhances electron mobility and conductivity while maintaining high transparency, making it suitable for ultrathin TCOs in flexible electronics. []
- Europium (Eu) doping: Improves photocatalytic activity for dye degradation under sunlight. []
Q14: How does the concentration of dopants affect In2O3's properties?
A14: The concentration of dopants plays a crucial role in tuning In2O3's properties. For instance, increasing the doping level of Zn in In2O3 can enhance electron mobility and conductivity up to an optimal point. Beyond this point, further doping may lead to a decrease in performance due to increased scattering effects. []
Q15: What are some common methods for synthesizing In2O3?
A15: * Sol-gel method: A versatile and cost-effective method for producing In2O3 nanoparticles. [, ]* Hydrothermal synthesis: Allows for controlled morphology and particle size of In2O3 nanostructures. [, , , , , , ]* Pulsed laser deposition (PLD): Enables the growth of high-quality thin films with controlled thickness and composition. []* Sputtering: Used for depositing thin films with good uniformity and controllable thickness. [, , , ]* Chemical vapor deposition (CVD): Suitable for large-area deposition of In2O3 thin films. [, ]* Electrospinning: Used for fabricating one-dimensional In2O3 nanofibers. []
Q16: How do different synthesis methods influence the properties of In2O3?
A16: Each synthesis method offers advantages and influences the final properties of In2O3. * Hydrothermal methods offer control over morphology, particle size, and crystallinity, impacting the material's photocatalytic and gas sensing properties. [, , , , , , ]* Sputtering techniques allow for precise control over film thickness and composition, influencing electrical conductivity and transparency in TCO applications. [, , , ]
Disclaimer and Information on In-Vitro Research Products
Please be aware that all articles and product information presented on BenchChem are intended solely for informational purposes. The products available for purchase on BenchChem are specifically designed for in-vitro studies, which are conducted outside of living organisms. In-vitro studies, derived from the Latin term "in glass," involve experiments performed in controlled laboratory settings using cells or tissues. It is important to note that these products are not categorized as medicines or drugs, and they have not received approval from the FDA for the prevention, treatment, or cure of any medical condition, ailment, or disease. We must emphasize that any form of bodily introduction of these products into humans or animals is strictly prohibited by law. It is essential to adhere to these guidelines to ensure compliance with legal and ethical standards in research and experimentation.