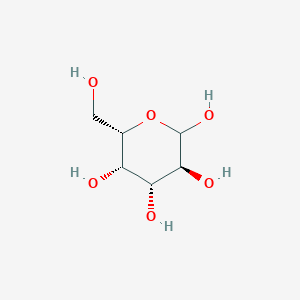
L-galactopyranose
Overview
Description
L-galactopyranose is a monosaccharide, specifically an aldohexose, which is a type of simple sugar. It is a stereoisomer of D-galactose and is commonly found in nature as part of various polysaccharides and glycoproteins. This compound is known for its role in the structure of agarose, a polysaccharide obtained from red algae, and is also a component of porphyran, another polysaccharide found in red algae .
Preparation Methods
Synthetic Routes and Reaction Conditions
L-galactopyranose can be synthesized through the hydrolysis of polysaccharides such as agarose and porphyran. Enzymatic hydrolysis using specific glycoside hydrolases like α-agarase and β-agarase can effectively break down these polysaccharides into their monosaccharide components, including this compound .
Industrial Production Methods
Industrial production of this compound typically involves the extraction and purification from natural sources such as red algae. The process includes the hydrolysis of agarose or porphyran using acid or enzymatic methods, followed by purification steps like activated carbon adsorption to remove impurities .
Chemical Reactions Analysis
Types of Reactions
L-galactopyranose undergoes various chemical reactions, including:
Oxidation: this compound can be oxidized to produce galactonic acid.
Reduction: It can be reduced to form galactitol.
Substitution: It can undergo substitution reactions to form esters and ethers
Common Reagents and Conditions
Oxidation: Common oxidizing agents include nitric acid and bromine water.
Reduction: Sodium borohydride and lithium aluminum hydride are typical reducing agents.
Substitution: Acylating agents like acetic anhydride and esterification catalysts such as DMAP (4-dimethylaminopyridine) are used.
Major Products
Oxidation: Galactonic acid.
Reduction: Galactitol.
Substitution: Various esters and ethers, depending on the reagents used
Scientific Research Applications
L-galactopyranose has numerous applications in scientific research:
Chemistry: Used as a building block in the synthesis of complex carbohydrates and glycosides.
Biology: Plays a role in the study of glycoproteins and glycolipids, which are essential for cell-cell communication and immune response.
Medicine: Investigated for its potential in drug delivery systems and as a component in antimicrobial and antiviral agents
Mechanism of Action
L-galactopyranose exerts its effects through various mechanisms:
Hydrogen Bonding: Forms strong hydrogen bonds, contributing to the gelation properties of agarose.
Enzymatic Interactions: Acts as a substrate for enzymes like α-agarase and β-agarase, which hydrolyze it into simpler sugars
Comparison with Similar Compounds
Similar Compounds
D-galactopyranose: The D-enantiomer of galactose, commonly found in lactose.
D-glucopyranose:
D-mannopyranose: An aldohexose similar to glucose but with a different stereochemistry at the C2 position
Uniqueness
L-galactopyranose is unique due to its specific stereochemistry, which allows it to form distinct polysaccharides like agarose and porphyran. These polysaccharides have unique properties, such as gelation and biological activity, making this compound valuable in various applications .
Properties
IUPAC Name |
(3S,4R,5S,6S)-6-(hydroxymethyl)oxane-2,3,4,5-tetrol | |
---|---|---|
Details | Computed by LexiChem 2.6.6 (PubChem release 2019.06.18) | |
Source | PubChem | |
URL | https://pubchem.ncbi.nlm.nih.gov | |
Description | Data deposited in or computed by PubChem | |
InChI |
InChI=1S/C6H12O6/c7-1-2-3(8)4(9)5(10)6(11)12-2/h2-11H,1H2/t2-,3+,4+,5-,6?/m0/s1 | |
Details | Computed by InChI 1.0.5 (PubChem release 2019.06.18) | |
Source | PubChem | |
URL | https://pubchem.ncbi.nlm.nih.gov | |
Description | Data deposited in or computed by PubChem | |
InChI Key |
WQZGKKKJIJFFOK-DHVFOXMCSA-N | |
Details | Computed by InChI 1.0.5 (PubChem release 2019.06.18) | |
Source | PubChem | |
URL | https://pubchem.ncbi.nlm.nih.gov | |
Description | Data deposited in or computed by PubChem | |
Canonical SMILES |
C(C1C(C(C(C(O1)O)O)O)O)O | |
Details | Computed by OEChem 2.1.5 (PubChem release 2019.06.18) | |
Source | PubChem | |
URL | https://pubchem.ncbi.nlm.nih.gov | |
Description | Data deposited in or computed by PubChem | |
Isomeric SMILES |
C([C@H]1[C@H]([C@H]([C@@H](C(O1)O)O)O)O)O | |
Details | Computed by OEChem 2.1.5 (PubChem release 2019.06.18) | |
Source | PubChem | |
URL | https://pubchem.ncbi.nlm.nih.gov | |
Description | Data deposited in or computed by PubChem | |
Molecular Formula |
C6H12O6 | |
Details | Computed by PubChem 2.1 (PubChem release 2019.06.18) | |
Source | PubChem | |
URL | https://pubchem.ncbi.nlm.nih.gov | |
Description | Data deposited in or computed by PubChem | |
DSSTOX Substance ID |
DTXSID901015855 | |
Record name | L-Galactopyranose | |
Source | EPA DSSTox | |
URL | https://comptox.epa.gov/dashboard/DTXSID901015855 | |
Description | DSSTox provides a high quality public chemistry resource for supporting improved predictive toxicology. | |
Molecular Weight |
180.16 g/mol | |
Details | Computed by PubChem 2.1 (PubChem release 2021.05.07) | |
Source | PubChem | |
URL | https://pubchem.ncbi.nlm.nih.gov | |
Description | Data deposited in or computed by PubChem | |
CAS No. |
39392-65-9 | |
Record name | L-Galactopyranose | |
Source | EPA DSSTox | |
URL | https://comptox.epa.gov/dashboard/DTXSID901015855 | |
Description | DSSTox provides a high quality public chemistry resource for supporting improved predictive toxicology. | |
Synthesis routes and methods I
Procedure details
Synthesis routes and methods II
Procedure details
Retrosynthesis Analysis
AI-Powered Synthesis Planning: Our tool employs the Template_relevance Pistachio, Template_relevance Bkms_metabolic, Template_relevance Pistachio_ringbreaker, Template_relevance Reaxys, Template_relevance Reaxys_biocatalysis model, leveraging a vast database of chemical reactions to predict feasible synthetic routes.
One-Step Synthesis Focus: Specifically designed for one-step synthesis, it provides concise and direct routes for your target compounds, streamlining the synthesis process.
Accurate Predictions: Utilizing the extensive PISTACHIO, BKMS_METABOLIC, PISTACHIO_RINGBREAKER, REAXYS, REAXYS_BIOCATALYSIS database, our tool offers high-accuracy predictions, reflecting the latest in chemical research and data.
Strategy Settings
Precursor scoring | Relevance Heuristic |
---|---|
Min. plausibility | 0.01 |
Model | Template_relevance |
Template Set | Pistachio/Bkms_metabolic/Pistachio_ringbreaker/Reaxys/Reaxys_biocatalysis |
Top-N result to add to graph | 6 |
Feasible Synthetic Routes
Disclaimer and Information on In-Vitro Research Products
Please be aware that all articles and product information presented on BenchChem are intended solely for informational purposes. The products available for purchase on BenchChem are specifically designed for in-vitro studies, which are conducted outside of living organisms. In-vitro studies, derived from the Latin term "in glass," involve experiments performed in controlled laboratory settings using cells or tissues. It is important to note that these products are not categorized as medicines or drugs, and they have not received approval from the FDA for the prevention, treatment, or cure of any medical condition, ailment, or disease. We must emphasize that any form of bodily introduction of these products into humans or animals is strictly prohibited by law. It is essential to adhere to these guidelines to ensure compliance with legal and ethical standards in research and experimentation.