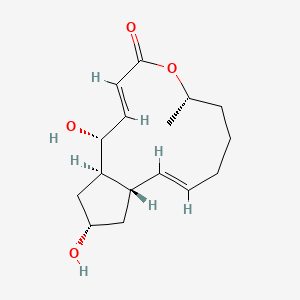
brefeldin A
Overview
Description
Brefeldin A is a lactone antiviral compound produced by the fungus Penicillium brefeldianum . It was initially isolated in 1958 from Penicillium decumbens and later identified as a metabolite by H.P. Siggs in 1971 . Although it was initially explored as an antiviral drug, it is now primarily used in research to study protein transport .
Preparation Methods
Synthetic Routes and Reaction Conditions: Brefeldin A can be synthesized through various methods. One common approach involves the use of nuclear magnetic resonance techniques, infrared spectrometry, electrospray ionization mass spectroscopy, and X-ray analysis to identify and synthesize the compound . The synthesis typically involves the formation of a macrocyclic lactone structure, which is a key feature of this compound .
Industrial Production Methods: Industrial production of this compound involves the cultivation of Penicillium brefeldianum or other related fungal species in liquid culture. The compound is then extracted and purified using various chromatographic techniques .
Chemical Reactions Analysis
Types of Reactions: Brefeldin A undergoes several types of chemical reactions, including oxidation, reduction, and substitution reactions .
Common Reagents and Conditions:
Oxidation: this compound can be oxidized using reagents such as hydrogen peroxide and ammonium molybdate.
Reduction: Reduction reactions can be carried out using reagents like lithium aluminum hydride.
Substitution: Substitution reactions often involve the use of triphenylphosphine and diethyl azodicarboxylate.
Major Products: The major products formed from these reactions include various analogs and derivatives of this compound, which are often explored for their potential biological activities .
Scientific Research Applications
Brefeldin A has a wide range of scientific research applications:
Mechanism of Action
Brefeldin A inhibits vesicle formation and transport between the endoplasmic reticulum and the Golgi apparatus, leading to the collapse of the Golgi apparatus into the endoplasmic reticulum via membrane fusion . It blocks the dissociation of activated STING (stimulator of interferon genes) from the endoplasmic reticulum, preventing the movement of STING to the endoplasmic reticulum-Golgi intermediate compartment, where it activates the TBK1-IRF3 signaling axis and triggers the expression of interferons .
Comparison with Similar Compounds
- Ascotoxin
- Cyanein
- Decumbin
- Nectrolide
- Synergisidin
Comparison: Brefeldin A is unique due to its potent inhibition of protein trafficking and its ability to induce apoptosis in cancer cells . While similar compounds like Ascotoxin and Cyanein also exhibit biological activities, this compound’s specific mechanism of action involving the disruption of the Golgi apparatus sets it apart .
Properties
IUPAC Name |
(1R,2R,3E,7S,11E,13S,15S)-2,15-dihydroxy-7-methyl-6-oxabicyclo[11.3.0]hexadeca-3,11-dien-5-one | |
---|---|---|
Source | PubChem | |
URL | https://pubchem.ncbi.nlm.nih.gov | |
Description | Data deposited in or computed by PubChem | |
InChI |
InChI=1S/C16H24O4/c1-11-5-3-2-4-6-12-9-13(17)10-14(12)15(18)7-8-16(19)20-11/h4,6-8,11-15,17-18H,2-3,5,9-10H2,1H3/b6-4+,8-7+/t11-,12+,13-,14+,15+/m0/s1 | |
Source | PubChem | |
URL | https://pubchem.ncbi.nlm.nih.gov | |
Description | Data deposited in or computed by PubChem | |
InChI Key |
KQNZDYYTLMIZCT-KQPMLPITSA-N | |
Source | PubChem | |
URL | https://pubchem.ncbi.nlm.nih.gov | |
Description | Data deposited in or computed by PubChem | |
Canonical SMILES |
CC1CCCC=CC2CC(CC2C(C=CC(=O)O1)O)O | |
Source | PubChem | |
URL | https://pubchem.ncbi.nlm.nih.gov | |
Description | Data deposited in or computed by PubChem | |
Isomeric SMILES |
C[C@H]1CCC/C=C/[C@@H]2C[C@@H](C[C@H]2[C@@H](/C=C/C(=O)O1)O)O | |
Source | PubChem | |
URL | https://pubchem.ncbi.nlm.nih.gov | |
Description | Data deposited in or computed by PubChem | |
Molecular Formula |
C16H24O4 | |
Source | PubChem | |
URL | https://pubchem.ncbi.nlm.nih.gov | |
Description | Data deposited in or computed by PubChem | |
DSSTOX Substance ID |
DTXSID00880041 | |
Record name | Brefeldin A | |
Source | EPA DSSTox | |
URL | https://comptox.epa.gov/dashboard/DTXSID00880041 | |
Description | DSSTox provides a high quality public chemistry resource for supporting improved predictive toxicology. | |
Molecular Weight |
280.36 g/mol | |
Source | PubChem | |
URL | https://pubchem.ncbi.nlm.nih.gov | |
Description | Data deposited in or computed by PubChem | |
CAS No. |
20350-15-6, 60132-23-2 | |
Record name | Brefeldin A | |
Source | CAS Common Chemistry | |
URL | https://commonchemistry.cas.org/detail?cas_rn=20350-15-6 | |
Description | CAS Common Chemistry is an open community resource for accessing chemical information. Nearly 500,000 chemical substances from CAS REGISTRY cover areas of community interest, including common and frequently regulated chemicals, and those relevant to high school and undergraduate chemistry classes. This chemical information, curated by our expert scientists, is provided in alignment with our mission as a division of the American Chemical Society. | |
Explanation | The data from CAS Common Chemistry is provided under a CC-BY-NC 4.0 license, unless otherwise stated. | |
Record name | Brefeldin A | |
Source | ChemIDplus | |
URL | https://pubchem.ncbi.nlm.nih.gov/substance/?source=chemidplus&sourceid=0020350156 | |
Description | ChemIDplus is a free, web search system that provides access to the structure and nomenclature authority files used for the identification of chemical substances cited in National Library of Medicine (NLM) databases, including the TOXNET system. | |
Record name | Brefeldin A | |
Source | DrugBank | |
URL | https://www.drugbank.ca/drugs/DB07348 | |
Description | The DrugBank database is a unique bioinformatics and cheminformatics resource that combines detailed drug (i.e. chemical, pharmacological and pharmaceutical) data with comprehensive drug target (i.e. sequence, structure, and pathway) information. | |
Explanation | Creative Common's Attribution-NonCommercial 4.0 International License (http://creativecommons.org/licenses/by-nc/4.0/legalcode) | |
Record name | brefeldin A | |
Source | DTP/NCI | |
URL | https://dtp.cancer.gov/dtpstandard/servlet/dwindex?searchtype=NSC&outputformat=html&searchlist=107456 | |
Description | The NCI Development Therapeutics Program (DTP) provides services and resources to the academic and private-sector research communities worldwide to facilitate the discovery and development of new cancer therapeutic agents. | |
Explanation | Unless otherwise indicated, all text within NCI products is free of copyright and may be reused without our permission. Credit the National Cancer Institute as the source. | |
Record name | brefeldin A | |
Source | DTP/NCI | |
URL | https://dtp.cancer.gov/dtpstandard/servlet/dwindex?searchtype=NSC&outputformat=html&searchlist=89671 | |
Description | The NCI Development Therapeutics Program (DTP) provides services and resources to the academic and private-sector research communities worldwide to facilitate the discovery and development of new cancer therapeutic agents. | |
Explanation | Unless otherwise indicated, all text within NCI products is free of copyright and may be reused without our permission. Credit the National Cancer Institute as the source. | |
Record name | brefeldin A | |
Source | DTP/NCI | |
URL | https://dtp.cancer.gov/dtpstandard/servlet/dwindex?searchtype=NSC&outputformat=html&searchlist=244390 | |
Description | The NCI Development Therapeutics Program (DTP) provides services and resources to the academic and private-sector research communities worldwide to facilitate the discovery and development of new cancer therapeutic agents. | |
Explanation | Unless otherwise indicated, all text within NCI products is free of copyright and may be reused without our permission. Credit the National Cancer Institute as the source. | |
Record name | brefeldin A | |
Source | DTP/NCI | |
URL | https://dtp.cancer.gov/dtpstandard/servlet/dwindex?searchtype=NSC&outputformat=html&searchlist=56310 | |
Description | The NCI Development Therapeutics Program (DTP) provides services and resources to the academic and private-sector research communities worldwide to facilitate the discovery and development of new cancer therapeutic agents. | |
Explanation | Unless otherwise indicated, all text within NCI products is free of copyright and may be reused without our permission. Credit the National Cancer Institute as the source. | |
Record name | Brefeldin A | |
Source | EPA DSSTox | |
URL | https://comptox.epa.gov/dashboard/DTXSID00880041 | |
Description | DSSTox provides a high quality public chemistry resource for supporting improved predictive toxicology. | |
Record name | BREFELDIN A | |
Source | FDA Global Substance Registration System (GSRS) | |
URL | https://gsrs.ncats.nih.gov/ginas/app/beta/substances/XG0D35F9K6 | |
Description | The FDA Global Substance Registration System (GSRS) enables the efficient and accurate exchange of information on what substances are in regulated products. Instead of relying on names, which vary across regulatory domains, countries, and regions, the GSRS knowledge base makes it possible for substances to be defined by standardized, scientific descriptions. | |
Explanation | Unless otherwise noted, the contents of the FDA website (www.fda.gov), both text and graphics, are not copyrighted. They are in the public domain and may be republished, reprinted and otherwise used freely by anyone without the need to obtain permission from FDA. Credit to the U.S. Food and Drug Administration as the source is appreciated but not required. | |
Retrosynthesis Analysis
AI-Powered Synthesis Planning: Our tool employs the Template_relevance Pistachio, Template_relevance Bkms_metabolic, Template_relevance Pistachio_ringbreaker, Template_relevance Reaxys, Template_relevance Reaxys_biocatalysis model, leveraging a vast database of chemical reactions to predict feasible synthetic routes.
One-Step Synthesis Focus: Specifically designed for one-step synthesis, it provides concise and direct routes for your target compounds, streamlining the synthesis process.
Accurate Predictions: Utilizing the extensive PISTACHIO, BKMS_METABOLIC, PISTACHIO_RINGBREAKER, REAXYS, REAXYS_BIOCATALYSIS database, our tool offers high-accuracy predictions, reflecting the latest in chemical research and data.
Strategy Settings
Precursor scoring | Relevance Heuristic |
---|---|
Min. plausibility | 0.01 |
Model | Template_relevance |
Template Set | Pistachio/Bkms_metabolic/Pistachio_ringbreaker/Reaxys/Reaxys_biocatalysis |
Top-N result to add to graph | 6 |
Feasible Synthetic Routes
Disclaimer and Information on In-Vitro Research Products
Please be aware that all articles and product information presented on BenchChem are intended solely for informational purposes. The products available for purchase on BenchChem are specifically designed for in-vitro studies, which are conducted outside of living organisms. In-vitro studies, derived from the Latin term "in glass," involve experiments performed in controlled laboratory settings using cells or tissues. It is important to note that these products are not categorized as medicines or drugs, and they have not received approval from the FDA for the prevention, treatment, or cure of any medical condition, ailment, or disease. We must emphasize that any form of bodily introduction of these products into humans or animals is strictly prohibited by law. It is essential to adhere to these guidelines to ensure compliance with legal and ethical standards in research and experimentation.