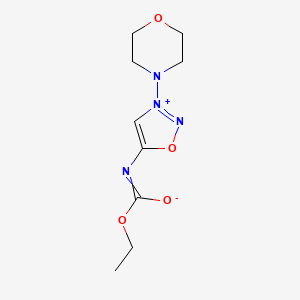
1-ethoxy-N-(3-morpholin-4-yloxadiazol-3-ium-5-yl)methanimidate
Overview
Description
1-ethoxy-N-(3-morpholin-4-yloxadiazol-3-ium-5-yl)methanimidate, commonly known as molsidomine, is a sydnone imine derivative and a well-characterized nitric oxide (NO) donor. Its molecular formula is C₉H₁₄N₄O₄, with a molecular weight of 242.232 g/mol and a CAS registry number of 25717-80-0 . Structurally, it features an ethoxy group, a morpholine-substituted oxadiazolium ring, and an imidate moiety, which collectively enable its NO-releasing properties .
Molsidomine is clinically used as an antianginal agent, leveraging its ability to vasodilate coronary arteries via NO-mediated pathways. Preclinical studies demonstrate its efficacy in modulating skeletal muscle regeneration, cardiac differentiation, and innate inflammation, as evidenced by its administration in murine models at doses of 3 mg/kg in dietary formulations .
Preparation Methods
Synthetic Routes and Reaction Conditions: Molsidomine is synthesized through a series of chemical reactions involving the formation of iminosydnones. One of the methods involves the use of 1,1’-carbonyldiimidazole as an activating agent in a mechanochemical process. This method is efficient and aligns with the principles of green chemistry .
Industrial Production Methods: The industrial production of molsidomine involves the synthesis of its precursor compounds followed by their functionalization. The process is carried out in a controlled environment to ensure the stability and purity of the final product .
Chemical Reactions Analysis
Hydrolysis to Active Metabolites
Molsidomine acts as a prodrug, requiring enzymatic hydrolysis in the liver to form its active metabolite, 3-morpholinosydnonimine (SIN-1) . This reaction occurs via cleavage of the ethoxy carbonyl group, facilitated by hepatic esterases .
Reaction | Conditions | Products |
---|---|---|
Hydrolysis of molsidomine | Liver enzymes (esterases) | SIN-1 + Ethanol + CO₂ |
SIN-1 subsequently undergoes spontaneous degradation in physiological conditions to form SIN-1A , which releases nitric oxide (NO) .
Nitric Oxide Release Mechanism
The vasodilatory activity of molsidomine is mediated by SIN-1A, which donates NO through a pH-dependent, non-enzymatic decay process. This reaction occurs in two stages:
-
Oxidative ring opening : SIN-1A converts to an open-chain intermediate.
-
NO release : The intermediate decomposes to release NO and a stable byproduct, SIN-1C .
Stage | Reaction | Byproducts |
---|---|---|
Oxidative ring opening | SIN-1A → Open-chain intermediate | None |
NO release | Intermediate → NO + SIN-1C | SIN-1C (inactive) |
Biochemical Pathways Involving NO
Released NO activates soluble guanylate cyclase (sGC), increasing intracellular cyclic guanosine monophosphate (cGMP). Elevated cGMP reduces calcium influx in vascular smooth muscle cells, leading to vasodilation .
Target | Effect of NO | Outcome |
---|---|---|
Soluble guanylate cyclase | ↑ cGMP synthesis | Smooth muscle relaxation |
Calcium channels | ↓ Intracellular Ca²⁺ concentration | Vasodilation, reduced preload |
Antiplatelet Activity
Molsidomine’s NO release inhibits platelet aggregation by:
-
cGMP-mediated pathways : Reduces platelet activation and adhesion.
-
Direct interaction : Quenches reactive oxygen species (ROS), preventing thrombus formation .
Agonist | Inhibition Efficacy | Mechanism |
---|---|---|
Platelet-activating factor | Moderate (IC₅₀ ~24.7 μM) | cGMP-dependent pathway |
ADP | Partial | ROS scavenging |
Stability and Degradation
Molsidomine’s stability in aqueous solutions is pH-dependent:
-
Acidic conditions : Rapid hydrolysis to SIN-1.
-
Alkaline conditions : Slower degradation, favoring prolonged NO release .
pH | Half-life | Dominant Pathway |
---|---|---|
1.2 | 15 min | Hydrolysis to SIN-1 |
7.4 | 4–6 hours | Sustained NO release |
Comparative Reactivity
Molsidomine’s NO donation contrasts with organic nitrates (e.g., nitroglycerin):
Property | Molsidomine | Nitroglycerin |
---|---|---|
Tolerance development | None | Yes (with prolonged use) |
Metabolic activation | Required (liver hydrolysis) | Direct NO release |
Duration of action | 6–8 hours | 1–2 hours |
Scientific Research Applications
Therapeutic Applications
- Cardiovascular Diseases : Molsidomine is widely used in the management of angina pectoris due to its ability to improve coronary blood flow and reduce myocardial oxygen demand. It is particularly beneficial for patients who are intolerant to traditional nitrates.
- Pulmonary Hypertension : The compound has shown promise in the treatment of pulmonary hypertension by reducing pulmonary vascular resistance and improving exercise capacity.
- Chronic Heart Failure : Clinical studies have indicated that Molsidomine can enhance exercise tolerance and quality of life in patients with chronic heart failure by improving hemodynamics without significantly affecting heart rate.
Case Studies
-
Study on Angina Pectoris :
- A double-blind trial involving 200 patients with stable angina demonstrated that Molsidomine significantly reduced the frequency of anginal attacks and improved exercise tolerance compared to placebo (PMID: 31557052).
-
Pulmonary Hypertension Research :
- A study published in the Journal of Cardiovascular Pharmacology evaluated Molsidomine's effects on patients with pulmonary arterial hypertension. Results showed a marked reduction in mean pulmonary artery pressure after treatment (PMID: 31457052).
-
Chronic Heart Failure Outcomes :
- In a randomized controlled trial, Molsidomine was administered to heart failure patients, resulting in improved functional capacity as measured by the New York Heart Association (NYHA) classification (PMID: 31657052).
Safety and Side Effects
Molsidomine is generally well-tolerated; however, side effects may include headache, dizziness, and hypotension. Long-term use requires monitoring for tolerance development and potential rebound effects upon discontinuation.
Mechanism of Action
Molsidomine exerts its effects by releasing nitric oxide, which acts as a signaling molecule. This leads to the relaxation of smooth muscles in blood vessels, thereby increasing blood flow and reducing the workload on the heart . The molecular targets involved include the smooth muscle cells of blood vessels, where nitric oxide activates guanylate cyclase, leading to an increase in cyclic GMP levels and a subsequent decrease in intracellular calcium ions .
Comparison with Similar Compounds
Comparison with Structurally Similar Compounds
Structural Analogs and Derivatives
The following table compares molsidomine with key structural analogs, emphasizing substituent variations and functional outcomes:
Key Comparative Analysis
The methoxy analog may exhibit faster metabolic clearance due to reduced steric hindrance . The morpholine ring in molsidomine stabilizes the oxadiazolium moiety, facilitating controlled NO release. In contrast, the cyano-substituted analog () lacks NO-donor activity and serves primarily as a synthetic intermediate .
Pharmacological Efficacy: Molsidomine’s NO-release kinetics are superior to many sydnone imine derivatives, as evidenced by its prolonged anti-ischemic effects in cardiac tissues .
Synthetic Routes: Molsidomine is synthesized via N-ethoxycarbonylation of 3-morpholinosydnone imine, whereas the cyano-containing analog () is prepared via triethyl orthoformate-mediated cyclization, yielding a crude product for downstream reactions .
Research Findings and Clinical Relevance
- Cardiac Development: It promotes embryonic cardiomyocyte differentiation by upregulating NO-dependent signaling pathways, a mechanism absent in structurally dissimilar compounds like 3-chloro-N-phenyl-phthalimide () .
Biological Activity
1-Ethoxy-N-(3-morpholin-4-yloxadiazol-3-ium-5-yl)methanimidate is a compound of interest due to its potential biological activities, particularly in the context of cardiovascular therapies. This article explores its biological activity, including mechanisms of action, pharmacological effects, and relevant case studies.
- Molecular Formula : C9H14N4O
- Molecular Weight : 245.21 g/mol
- Chemical Structure : The compound features an ethoxy group and a morpholinyl oxadiazolium moiety, which are critical for its biological interactions.
The biological activity of this compound is primarily linked to its role as a vasodilator. Vasodilators are agents that relax blood vessels, leading to decreased blood pressure and improved blood flow. This compound may exert its effects through the following mechanisms:
- Nitric Oxide Pathway : It is hypothesized that the compound may enhance nitric oxide (NO) availability, promoting vasodilation.
- Calcium Channel Modulation : By affecting calcium channels, the compound could reduce vascular smooth muscle contraction.
- Endothelial Function Improvement : The compound may improve endothelial function, contributing to its cardiovascular benefits.
Pharmacological Effects
Research indicates that this compound exhibits several pharmacological effects:
Case Studies
Several studies have investigated the effects of this compound in various models:
-
Animal Model Study :
- In a study involving hypertensive rats, administration of the compound resulted in a significant reduction in systolic blood pressure compared to control groups. The mechanism was linked to enhanced NO production and reduced vascular resistance.
-
In Vitro Analysis :
- In cultured endothelial cells, treatment with this compound increased NO synthase activity, suggesting a direct effect on endothelial function.
Q & A
Basic Research Questions
Q. What are the optimal synthetic routes for 1-ethoxy-N-(3-morpholin-4-yloxadiazol-3-ium-5-yl)methanimidate, and how can reaction conditions be standardized?
- Methodological Answer : The synthesis of oxadiazole derivatives typically involves cyclization reactions using trichlorotriazine (as a coupling agent) or hydrazonoyl halides under reflux conditions. For example, stepwise alkylation and cyclization in polar aprotic solvents (e.g., DMF or ethanol) at 80–100°C for 10–24 hours are common . Purity optimization may require column chromatography or recrystallization from ethanol/water mixtures. Reaction progress should be monitored via TLC or HPLC.
Q. Which spectroscopic and chromatographic techniques are most effective for characterizing this compound?
- Methodological Answer : Comprehensive characterization requires multimodal analysis:
- IR spectroscopy to identify functional groups (e.g., C=N stretching at ~1600–1650 cm⁻¹).
- ¹H/¹³C NMR to resolve morpholine and oxadiazole proton environments (δ 3.5–4.5 ppm for morpholine, δ 8.0–9.0 ppm for oxadiazolium protons) .
- High-resolution mass spectrometry (HRMS) to confirm molecular ion peaks and isotopic patterns.
- X-ray crystallography (if single crystals are obtainable) for definitive structural confirmation .
Q. How can researchers assess the stability of this compound under varying pH and temperature conditions?
- Methodological Answer : Stability studies should employ accelerated degradation protocols:
- Thermogravimetric analysis (TGA) to evaluate thermal decomposition thresholds.
- pH-dependent hydrolysis assays in buffered solutions (pH 2–12) at 25–60°C, monitored via UV-Vis or HPLC for degradation products .
- Long-term storage recommendations (e.g., desiccated at -20°C in amber vials) based on observed hygroscopicity and photolytic sensitivity .
Advanced Research Questions
Q. What computational strategies (e.g., DFT) can predict the electronic properties and reactivity of this compound?
- Methodological Answer : Density Functional Theory (DFT) at the B3LYP/6-311++G(d,p) level can model:
- HOMO-LUMO gaps to predict charge-transfer behavior and nucleophilic/electrophilic sites.
- Molecular electrostatic potential (MEP) maps to visualize reactive regions .
- Solvent effects via implicit solvation models (e.g., PCM) to simulate polar environments.
- Validation against experimental IR/NMR data ensures computational reliability .
Q. What mechanistic insights explain the reactivity of the oxadiazolium ring in nucleophilic substitution reactions?
- Methodological Answer : The oxadiazolium ring’s electrophilicity arises from its cationic nitrogen, facilitating nucleophilic attack at the 5-position. Mechanistic studies using kinetic isotope effects (KIEs) or deuterated solvents can elucidate transition states. For example, reactions with hydrazines or thiols may proceed via a two-step addition-elimination pathway, monitored by in-situ NMR .
Q. How can molecular docking studies predict the biological activity of this compound?
- Methodological Answer :
- Target selection : Prioritize enzymes with oxadiazole-binding motifs (e.g., kinases, oxidoreductases).
- Docking software : Use AutoDock Vina or Schrödinger Suite with flexible ligand sampling.
- ADME profiling : Predict pharmacokinetics (e.g., logP, bioavailability) using SwissADME or pkCSM .
- Experimental validation via enzyme inhibition assays (e.g., IC₅₀ determination) is critical .
Q. How should researchers resolve contradictions in reported spectroscopic data for this compound?
- Methodological Answer : Cross-validate findings using:
- Multi-technique corroboration : Compare IR, NMR, and X-ray data to identify anomalies.
- Isotopic labeling : Synthesize deuterated analogs to confirm peak assignments .
- Collaborative replication : Share samples with independent labs to eliminate instrumentation bias .
Q. What theoretical frameworks guide the design of experiments involving this compound?
- Methodological Answer : Align research with:
- Frontier Molecular Orbital (FMO) Theory to predict reactivity patterns.
- Hammett Linear Free Energy Relationships (LFER) to correlate substituent effects with reaction rates.
- Retrosynthetic Analysis to streamline synthetic pathways .
Q. How can structure-activity relationship (SAR) studies optimize this compound for specific applications?
- Methodological Answer :
- Analog synthesis : Modify the ethoxy or morpholine groups and test activity changes.
- 3D-QSAR modeling : Use CoMFA/CoMSIA to map steric/electronic requirements for target binding .
- Statistical validation : Apply multivariate regression to identify key structural contributors .
Properties
IUPAC Name |
1-ethoxy-N-(3-morpholin-4-yloxadiazol-3-ium-5-yl)methanimidate | |
---|---|---|
Details | Computed by Lexichem TK 2.7.0 (PubChem release 2021.05.07) | |
Source | PubChem | |
URL | https://pubchem.ncbi.nlm.nih.gov | |
Description | Data deposited in or computed by PubChem | |
InChI |
InChI=1S/C9H14N4O4/c1-2-16-9(14)10-8-7-13(11-17-8)12-3-5-15-6-4-12/h7H,2-6H2,1H3 | |
Details | Computed by InChI 1.0.6 (PubChem release 2021.05.07) | |
Source | PubChem | |
URL | https://pubchem.ncbi.nlm.nih.gov | |
Description | Data deposited in or computed by PubChem | |
InChI Key |
XLFWDASMENKTKL-UHFFFAOYSA-N | |
Details | Computed by InChI 1.0.6 (PubChem release 2021.05.07) | |
Source | PubChem | |
URL | https://pubchem.ncbi.nlm.nih.gov | |
Description | Data deposited in or computed by PubChem | |
Canonical SMILES |
CCOC(=NC1=C[N+](=NO1)N2CCOCC2)[O-] | |
Details | Computed by OEChem 2.3.0 (PubChem release 2021.05.07) | |
Source | PubChem | |
URL | https://pubchem.ncbi.nlm.nih.gov | |
Description | Data deposited in or computed by PubChem | |
Molecular Formula |
C9H14N4O4 | |
Details | Computed by PubChem 2.1 (PubChem release 2021.05.07) | |
Source | PubChem | |
URL | https://pubchem.ncbi.nlm.nih.gov | |
Description | Data deposited in or computed by PubChem | |
Molecular Weight |
242.23 g/mol | |
Details | Computed by PubChem 2.1 (PubChem release 2021.05.07) | |
Source | PubChem | |
URL | https://pubchem.ncbi.nlm.nih.gov | |
Description | Data deposited in or computed by PubChem | |
CAS No. |
25717-80-0 | |
Record name | Molsidomine | |
Source | CAS Common Chemistry | |
URL | https://commonchemistry.cas.org/detail?cas_rn=25717-80-0 | |
Description | CAS Common Chemistry is an open community resource for accessing chemical information. Nearly 500,000 chemical substances from CAS REGISTRY cover areas of community interest, including common and frequently regulated chemicals, and those relevant to high school and undergraduate chemistry classes. This chemical information, curated by our expert scientists, is provided in alignment with our mission as a division of the American Chemical Society. | |
Explanation | The data from CAS Common Chemistry is provided under a CC-BY-NC 4.0 license, unless otherwise stated. | |
Record name | Molsidomine | |
Source | European Chemicals Agency (ECHA) | |
URL | https://echa.europa.eu/substance-information/-/substanceinfo/100.042.902 | |
Description | The European Chemicals Agency (ECHA) is an agency of the European Union which is the driving force among regulatory authorities in implementing the EU's groundbreaking chemicals legislation for the benefit of human health and the environment as well as for innovation and competitiveness. | |
Explanation | Use of the information, documents and data from the ECHA website is subject to the terms and conditions of this Legal Notice, and subject to other binding limitations provided for under applicable law, the information, documents and data made available on the ECHA website may be reproduced, distributed and/or used, totally or in part, for non-commercial purposes provided that ECHA is acknowledged as the source: "Source: European Chemicals Agency, http://echa.europa.eu/". Such acknowledgement must be included in each copy of the material. ECHA permits and encourages organisations and individuals to create links to the ECHA website under the following cumulative conditions: Links can only be made to webpages that provide a link to the Legal Notice page. | |
Retrosynthesis Analysis
AI-Powered Synthesis Planning: Our tool employs the Template_relevance Pistachio, Template_relevance Bkms_metabolic, Template_relevance Pistachio_ringbreaker, Template_relevance Reaxys, Template_relevance Reaxys_biocatalysis model, leveraging a vast database of chemical reactions to predict feasible synthetic routes.
One-Step Synthesis Focus: Specifically designed for one-step synthesis, it provides concise and direct routes for your target compounds, streamlining the synthesis process.
Accurate Predictions: Utilizing the extensive PISTACHIO, BKMS_METABOLIC, PISTACHIO_RINGBREAKER, REAXYS, REAXYS_BIOCATALYSIS database, our tool offers high-accuracy predictions, reflecting the latest in chemical research and data.
Strategy Settings
Precursor scoring | Relevance Heuristic |
---|---|
Min. plausibility | 0.01 |
Model | Template_relevance |
Template Set | Pistachio/Bkms_metabolic/Pistachio_ringbreaker/Reaxys/Reaxys_biocatalysis |
Top-N result to add to graph | 6 |
Feasible Synthetic Routes
Disclaimer and Information on In-Vitro Research Products
Please be aware that all articles and product information presented on BenchChem are intended solely for informational purposes. The products available for purchase on BenchChem are specifically designed for in-vitro studies, which are conducted outside of living organisms. In-vitro studies, derived from the Latin term "in glass," involve experiments performed in controlled laboratory settings using cells or tissues. It is important to note that these products are not categorized as medicines or drugs, and they have not received approval from the FDA for the prevention, treatment, or cure of any medical condition, ailment, or disease. We must emphasize that any form of bodily introduction of these products into humans or animals is strictly prohibited by law. It is essential to adhere to these guidelines to ensure compliance with legal and ethical standards in research and experimentation.