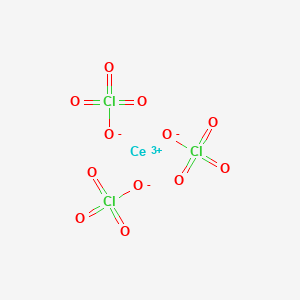
Cerium(3+) perchlorate
- Click on QUICK INQUIRY to receive a quote from our team of experts.
- With the quality product at a COMPETITIVE price, you can focus more on your research.
Overview
Description
Cerium(3+) perchlorate is an inorganic compound composed of cerium and perchloric acid. It is a cerium salt where cerium is in the +3 oxidation state. This compound is known for its high solubility in water and its use in various chemical applications due to its unique properties.
Scientific Research Applications
Cerium(3+) perchlorate has a wide range of applications in scientific research:
Chemistry: Used as a catalyst in organic synthesis and as a reagent in various chemical reactions.
Biology: Investigated for its potential use in biological systems due to its redox properties.
Medicine: Studied for its potential therapeutic applications, including its use in wound healing and as an antimicrobial agent.
Industry: Utilized in the production of advanced materials and as a component in various industrial processes.
Mechanism of Action
Target of Action
Cerium(3+) perchlorate, also known as cerium(3+);triperchlorate, primarily targets formamidine derivatives . It is used as a catalyst in organic chemistry for the determination of strontium and for cerimetry .
Mode of Action
The compound interacts with its targets through a process of oxidation . The kinetics of oxidation of formamidine derivatives by cerium(IV) has been investigated in aqueous perchlorate solutions . The reaction exhibited a first-order kinetics with respect to [Ce (IV)], whereas the order with respect to [formamidine derivative] was found to be less than unity . The plausible oxidation mechanism involves the formation of an intermediate complex between the formamidine substrate and the kinetically active species of cerium(IV) in a pre-equilibrium step which decomposes in the slow step to give a free radical derived from the substrate and Ce (III) .
Biochemical Pathways
The biochemical pathways affected by this compound involve the oxidation of dicarboxylic acids . This process is part of the Belousov–Zhabotinsky oscillating reaction (BZ reaction) catalyzed by cerium ions . The reaction involves two parallel pathways, for which two different cerium(IV)–oxalate intermediate complexes were identified and characterized .
Pharmacokinetics
The reaction rate was found to be unaffected by increasing either ionic strength or dielectric constant of the medium . This suggests that the compound’s ADME properties and bioavailability may be influenced by these factors.
Result of Action
The result of the action of this compound is the formation of cerium(IV) dioxide . The thermal decomposition of cerium(III) perchlorate hydrate at 460°C leads to the formation of nanocrystalline cerium dioxide with a particle size of 13 nm .
Action Environment
The action of this compound is influenced by environmental factors such as temperature and ionic strength . The reaction rate was found to be unaffected by increasing either ionic strength or dielectric constant of the medium . Furthermore, the thermal decomposition of cerium(III) perchlorate hydrate at 460°C leads to the formation of nanocrystalline cerium dioxide .
Safety and Hazards
Future Directions
Future research directions for Cerium(3+) perchlorate could involve further development of La-based adsorbents for phosphate removal and recovery, direct comparisons among La compounds using more complex matrices such as wastewater and river water, and more pilot-scale studies involving La-based adsorbents . More detailed information about the future directions can be found in the referenced papers .
Biochemical Analysis
Biochemical Properties
Cerium compounds have been shown to interact with various biomolecules, potentially influencing biochemical reactions
Cellular Effects
Cerium compounds, including Cerium(3+) perchlorate, can have significant effects on cells. For instance, cerium oxide nanoparticles have shown promise as antioxidants in cell culture models and animal models of disease
Molecular Mechanism
Cerium compounds have been shown to exhibit redox behavior, which could influence their interactions with biomolecules
Temporal Effects in Laboratory Settings
One study has shown that the thermal decomposition of cerium(III) perchlorate hydrate at 460°C leads to the formation of nanocrystalline cerium dioxide .
Metabolic Pathways
Cerium compounds have been implicated in various biochemical processes
Transport and Distribution
Cerium compounds have been shown to interact with various cellular components, suggesting that this compound could also have specific transport and distribution patterns .
Subcellular Localization
Studies have shown that cerium oxide nanoparticles can localize to different cell compartments depending on the nanoparticle’s surface charge
Preparation Methods
Synthetic Routes and Reaction Conditions: Cerium(3+) perchlorate can be synthesized by reacting cerium(3+) oxide or cerium(3+) hydroxide with perchloric acid. The reaction typically involves dissolving cerium(3+) oxide or hydroxide in an aqueous solution of perchloric acid, followed by evaporation to obtain the crystalline product.
Industrial Production Methods: In industrial settings, this compound is often produced by the controlled reaction of cerium(3+) nitrate with perchloric acid. The process involves careful handling of perchloric acid due to its highly reactive and potentially explosive nature.
Chemical Reactions Analysis
Types of Reactions: Cerium(3+) perchlorate undergoes various chemical reactions, including:
Oxidation: Cerium(3+) can be oxidized to cerium(4+) in the presence of strong oxidizing agents.
Reduction: Cerium(4+) can be reduced back to cerium(3+) using reducing agents.
Substitution: this compound can participate in substitution reactions where the perchlorate ion is replaced by other anions.
Common Reagents and Conditions:
Oxidation: Strong oxidizing agents such as potassium permanganate or hydrogen peroxide.
Reduction: Reducing agents like sodium borohydride or hydrazine.
Substitution: Various anions like chloride, nitrate, or sulfate can replace the perchlorate ion under appropriate conditions.
Major Products:
Oxidation: Cerium(4+) compounds such as cerium(4+) oxide.
Reduction: Cerium(3+) compounds such as cerium(3+) chloride.
Substitution: Cerium salts with different anions, such as cerium(3+) chloride or cerium(3+) nitrate.
Comparison with Similar Compounds
Cerium(3+) chloride: Another cerium(3+) salt, known for its use in organic synthesis and as a precursor for other cerium compounds.
Cerium(3+) nitrate: Used in similar applications as cerium(3+) perchlorate, including as a catalyst and in material science.
Cerium(4+) perchlorate: A cerium(4+) salt with higher oxidation potential, used in different chemical reactions compared to this compound.
Uniqueness: this compound is unique due to its high solubility in water and its ability to participate in a wide range of chemical reactions. Its redox properties make it a valuable compound in both research and industrial applications.
Properties
{ "Design of the Synthesis Pathway": "The synthesis of Cerium(3+) perchlorate can be achieved by reacting cerium oxide or cerium nitrate with perchloric acid.", "Starting Materials": ["Cerium oxide or cerium nitrate", "Perchloric acid"], "Reaction": ["Dissolve cerium oxide or cerium nitrate in water to form cerium solution", "Add perchloric acid to the cerium solution slowly while stirring constantly", "Heat the reaction mixture to evaporate excess water and concentrate the solution", "Cool the solution to room temperature to allow Cerium(3+) perchlorate to crystallize out", "Filter the solid product and wash with cold water to remove any impurities", "Dry the product in a desiccator or vacuum oven at low temperature"], } | |
CAS No. |
14017-47-1 |
Molecular Formula |
CeClHO4 |
Molecular Weight |
240.57 g/mol |
IUPAC Name |
cerium;perchloric acid |
InChI |
InChI=1S/Ce.ClHO4/c;2-1(3,4)5/h;(H,2,3,4,5) |
InChI Key |
XKVZLRDEADGMFT-UHFFFAOYSA-N |
SMILES |
[O-]Cl(=O)(=O)=O.[O-]Cl(=O)(=O)=O.[O-]Cl(=O)(=O)=O.[Ce+3] |
Canonical SMILES |
OCl(=O)(=O)=O.[Ce] |
14017-47-1 | |
Pictograms |
Oxidizer; Irritant |
Origin of Product |
United States |
Retrosynthesis Analysis
AI-Powered Synthesis Planning: Our tool employs the Template_relevance Pistachio, Template_relevance Bkms_metabolic, Template_relevance Pistachio_ringbreaker, Template_relevance Reaxys, Template_relevance Reaxys_biocatalysis model, leveraging a vast database of chemical reactions to predict feasible synthetic routes.
One-Step Synthesis Focus: Specifically designed for one-step synthesis, it provides concise and direct routes for your target compounds, streamlining the synthesis process.
Accurate Predictions: Utilizing the extensive PISTACHIO, BKMS_METABOLIC, PISTACHIO_RINGBREAKER, REAXYS, REAXYS_BIOCATALYSIS database, our tool offers high-accuracy predictions, reflecting the latest in chemical research and data.
Strategy Settings
Precursor scoring | Relevance Heuristic |
---|---|
Min. plausibility | 0.01 |
Model | Template_relevance |
Template Set | Pistachio/Bkms_metabolic/Pistachio_ringbreaker/Reaxys/Reaxys_biocatalysis |
Top-N result to add to graph | 6 |
Feasible Synthetic Routes
Disclaimer and Information on In-Vitro Research Products
Please be aware that all articles and product information presented on BenchChem are intended solely for informational purposes. The products available for purchase on BenchChem are specifically designed for in-vitro studies, which are conducted outside of living organisms. In-vitro studies, derived from the Latin term "in glass," involve experiments performed in controlled laboratory settings using cells or tissues. It is important to note that these products are not categorized as medicines or drugs, and they have not received approval from the FDA for the prevention, treatment, or cure of any medical condition, ailment, or disease. We must emphasize that any form of bodily introduction of these products into humans or animals is strictly prohibited by law. It is essential to adhere to these guidelines to ensure compliance with legal and ethical standards in research and experimentation.