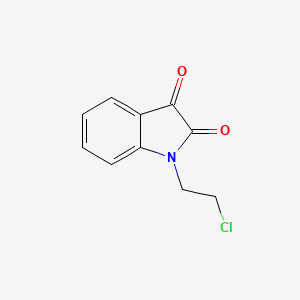
1-(2-chloroethyl)-1H-indole-2,3-dione
- Click on QUICK INQUIRY to receive a quote from our team of experts.
- With the quality product at a COMPETITIVE price, you can focus more on your research.
Overview
Description
1-(2-chloroethyl)-1H-indole-2,3-dione is a chemical compound that belongs to the class of indole derivatives Indole derivatives are significant in various fields due to their diverse biological and chemical properties
Preparation Methods
Synthetic Routes and Reaction Conditions
The synthesis of 1-(2-chloroethyl)-1H-indole-2,3-dione typically involves the reaction of indole-2,3-dione with 2-chloroethylamine under controlled conditions. The reaction is usually carried out in the presence of a suitable solvent, such as ethanol or acetonitrile, and may require a catalyst to facilitate the reaction. The reaction conditions, including temperature and time, are optimized to achieve high yield and purity of the desired product .
Industrial Production Methods
Industrial production of this compound may involve large-scale synthesis using continuous flow reactors. These reactors allow for precise control over reaction parameters, ensuring consistent product quality. The use of automated systems and advanced purification techniques, such as chromatography, further enhances the efficiency of the production process .
Chemical Reactions Analysis
Types of Reactions
1-(2-chloroethyl)-1H-indole-2,3-dione undergoes various chemical reactions, including:
Oxidation: The compound can be oxidized to form corresponding oxides.
Reduction: Reduction reactions can convert the compound into its reduced forms.
Substitution: The chloroethyl group can be substituted with other functional groups through nucleophilic substitution reactions.
Common Reagents and Conditions
Oxidation: Common oxidizing agents include potassium permanganate and hydrogen peroxide.
Reduction: Reducing agents such as sodium borohydride and lithium aluminum hydride are used.
Substitution: Nucleophiles like amines and thiols are employed in substitution reactions.
Major Products Formed
The major products formed from these reactions depend on the specific reagents and conditions used. For example, oxidation may yield oxides, while substitution reactions can produce a variety of substituted indole derivatives .
Scientific Research Applications
1-(2-chloroethyl)-1H-indole-2,3-dione has several scientific research applications:
Chemistry: It is used as a building block in the synthesis of complex organic molecules.
Biology: The compound is studied for its potential biological activities, including antimicrobial and anticancer properties.
Medicine: Research is ongoing to explore its potential as a therapeutic agent for various diseases.
Industry: It is used in the development of advanced materials and chemical sensors
Mechanism of Action
The mechanism of action of 1-(2-chloroethyl)-1H-indole-2,3-dione involves its interaction with specific molecular targets. The chloroethyl group can form covalent bonds with nucleophilic sites in biological molecules, leading to the inhibition of key enzymes and disruption of cellular processes. This mechanism is similar to that of other alkylating agents used in chemotherapy .
Comparison with Similar Compounds
Similar Compounds
Mechlorethamine: An alkylating agent used in chemotherapy.
Semustine: Another alkylating agent with similar properties.
Chlorambucil: Used in the treatment of chronic lymphocytic leukemia .
Uniqueness
1-(2-chloroethyl)-1H-indole-2,3-dione is unique due to its indole core structure, which imparts distinct chemical and biological properties.
Properties
IUPAC Name |
1-(2-chloroethyl)indole-2,3-dione |
Source
|
---|---|---|
Details | Computed by Lexichem TK 2.7.0 (PubChem release 2021.05.07) | |
Source | PubChem | |
URL | https://pubchem.ncbi.nlm.nih.gov | |
Description | Data deposited in or computed by PubChem | |
InChI |
InChI=1S/C10H8ClNO2/c11-5-6-12-8-4-2-1-3-7(8)9(13)10(12)14/h1-4H,5-6H2 |
Source
|
Details | Computed by InChI 1.0.6 (PubChem release 2021.05.07) | |
Source | PubChem | |
URL | https://pubchem.ncbi.nlm.nih.gov | |
Description | Data deposited in or computed by PubChem | |
InChI Key |
YWYAMZMSYNHYFZ-UHFFFAOYSA-N |
Source
|
Details | Computed by InChI 1.0.6 (PubChem release 2021.05.07) | |
Source | PubChem | |
URL | https://pubchem.ncbi.nlm.nih.gov | |
Description | Data deposited in or computed by PubChem | |
Canonical SMILES |
C1=CC=C2C(=C1)C(=O)C(=O)N2CCCl |
Source
|
Details | Computed by OEChem 2.3.0 (PubChem release 2021.05.07) | |
Source | PubChem | |
URL | https://pubchem.ncbi.nlm.nih.gov | |
Description | Data deposited in or computed by PubChem | |
Molecular Formula |
C10H8ClNO2 |
Source
|
Details | Computed by PubChem 2.1 (PubChem release 2021.05.07) | |
Source | PubChem | |
URL | https://pubchem.ncbi.nlm.nih.gov | |
Description | Data deposited in or computed by PubChem | |
Molecular Weight |
209.63 g/mol |
Source
|
Details | Computed by PubChem 2.1 (PubChem release 2021.05.07) | |
Source | PubChem | |
URL | https://pubchem.ncbi.nlm.nih.gov | |
Description | Data deposited in or computed by PubChem | |
Disclaimer and Information on In-Vitro Research Products
Please be aware that all articles and product information presented on BenchChem are intended solely for informational purposes. The products available for purchase on BenchChem are specifically designed for in-vitro studies, which are conducted outside of living organisms. In-vitro studies, derived from the Latin term "in glass," involve experiments performed in controlled laboratory settings using cells or tissues. It is important to note that these products are not categorized as medicines or drugs, and they have not received approval from the FDA for the prevention, treatment, or cure of any medical condition, ailment, or disease. We must emphasize that any form of bodily introduction of these products into humans or animals is strictly prohibited by law. It is essential to adhere to these guidelines to ensure compliance with legal and ethical standards in research and experimentation.